Radiation astronomy/Active galactic nuclei
{{fairuse}}
There is "a correlation between the arrival directions of cosmic rays with energy above 6 x 1019 electron volts and the positions of active galactic nuclei (AGN) lying within ~75 megaparsecs."[1]
Some low energy cosmic rays originate or are associated with solar flares. Even these cosmic rays have too high an energy to originate from the solar photosphere. The coronal cloud in close proximity to the Sun may be a source or create them as it bombards the chromosphere from above.
"In particular we recognize a first trace of Vela, brightest gamma and radio galactic source, and smeared sources along Galactic Plane and Center [as a source of ultra high energy cosmic rays (UHECR)]."[2]
"The main correlated map is the 408 MHz one. The first astronomical source that seem to correlate is the main multiplet along CenA. This AGN source, the nearest extragalactic one, sits in the same direction of a far Centaurus Cluster (part of the Super-Galactic Plane). The blurring by random galactic magnetic field might spread the nearest AGN event along the same Super-Galactic Plane, explaining the AUGER group miss-understanding [3]."[2]
Emissions[edit]
{{fairuse}}
"Seyfert galaxies were originally noted for the strength and broadening of their emission lines, and as a class were later characterized by the high ionization states of many of the atomic and ionized species producing these lines. This composite spectrum of the archetypal Seyfert NGC 4151 shows the wide variety of emission lines present, from the Lyman limit at 912 A to the mid-infrared at about 9 microns. It uses spectra taken with apertures several arcseconds in size, so as to reproduce the usual spectrum mixing broad and narrow-line components. From 912-1800 A, the data come from the Shuttle-borne Hopkins Ultraviolet Telescope; from 1800-3200 A, from the mean of three measurements by the International Ultraviolet Explorer (IUE) taken at similar brightness levels; from 3200-4000A, from an observation at Kitt Peak National Observatory, with the continuum rescaled to match the adjacent spectra; from 4000-8000 A, a CCD observation obtained at the Lick Observatory 3-m Shane telescope by Alexei Filippenko; from 8000 A to 1 microns, an observation using the same telescope by Donald Osterbrock and collaborators, carefully corrected for atmospheric absorption; from 0.9-2.4 microns, measurements by Rodger Thompson at Steward Observatory's 2,3-m Bok telescope, and on into the infrared, from the Infrared Space Observatory provided by Eckhard Sturm. Because NGC 4151 is irregularly variable, some of the spectral components have been scaled to make the various pieces match for this presentation (so the relative strengths of lines in very different spectral regions may not be accurate)."[3]
"Some of the most prominent emission lines are marked for reference. The permitted lines - those that can be produced at high densities by astronomical standards - show both brad and narrow components. The strongest of these are the hydrogen recombination lines, such as Lyman alpha at 1216 A, H-beta at 4861, and H-alpha at 6563, plus the strong ultraviolet lines of C IV at 1549 and Mg II at 2800. Other features produced only by very rarefied gas at densities of 1000 atoms per cubic centimeter or so - the forbidden lines, denoted by brackets - arise in regions with less velocity structure and are narrower. Some strong examples are [O III] at 4959 and 5007 A, [O II] at 3727, [Ne V] at 3426, and [S III] at 9060 and 9532."[3]
"The spectra of active galactic nuclei are noteworthy in showing species with a large range in ionization at once, from neutral ions such as [O I] and [N I] to highly ionized cases such as [Ne V] and [O VI]. Even hot stars such as light up gaseous nebulae in our galaxy cannot ionize gas as highly as these ions require, so that both a strong source of hard radiation and a wide range in gas density must be present to see such spectra."[3]
Standard candles[edit]
{{fairuse}}
“Accurate distances to celestial objects are key to establishing the age and energy density of the Universe and the nature of dark energy.”[4]
“A distance measure using active galactic nuclei (AGN) has been sought for more than forty years, as they are extremely luminous and can be observed at very large distances.”[4]
Active "galactic nuclei are home to supermassive black holes which unleash powerful radiation. When this radiation ionizes nearby gas clouds, they also emit their own light signature. With both emissions in range of data gathering telescopes, all that’s needed is a way to measure the time it takes between the radiation signal and the ionization point. The process is called reverberation mapping."[5]
“We use the tight relationship between the luminosity of an AGN and the radius of its broad line region established via reverberation mapping to determine the luminosity distances to a sample of 38 AGN.”[4]
“All reliable distance measures up to now have been limited to moderate redshift — AGN will, for the first time, allow distances to be estimated to z~4, where variations of dark energy and alternate gravity theories can be probed.”[4]
"The AGN Hubble diagram [is at the right]. The luminosity distance indicator τ is plotted as a function of redshift for 38 AGN with H lag measurements. On the right axis the luminosity distance and distance modulus (m-M) are shown using the surface brightness fluctuations distance to NGC3227 as a calibrator. The current best cosmology is plotted as a solid line. The line is not fit to the data but clearly follows the data well. Cosmologies with no dark energy components are plotted as dashed and dotted lines. The lower panel shows the logarithm of the ratio of the data compared to the current cosmology on the left axis, with the same values but in magnitudes on the right. The red arrow indicates the correction for internal extinction for NGC3516. The green arrow shows where NGC7469 would lie using the revised lag estimate. NGC7469 is our largest outlier and is believed to be an example of an object with a misidentified lag."[5]
“The scatter due to observational uncertainty can be reduced significantly. A major advantage held by AGN is that they can be observed repeatedly and the distance to any given object substantially refined.”[4]
“The ultimate limit of the accuracy of the method will rely on how the BLR (broad-line emitting region) responds to changes in the luminosity of the central source. The current tight radius-luminosity relationship indicates that the ionisation parameter and the gas density are both close to constant across our sample.”[4]
Continua[edit]
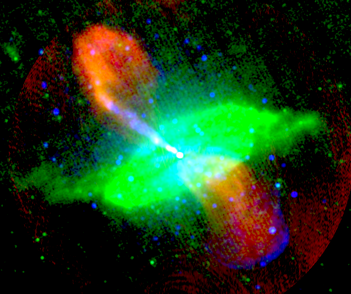
{{free media}}
The radio continuum from an active galactic nucleus is always due to a jet. It shows a spectrum characteristic of synchrotron radiation.
BL Lacertae objects[edit]
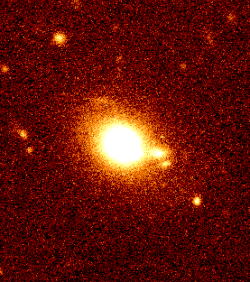
{{free media}}
A BL Lacertae object or BL Lac object is a type of active galaxy with an active galactic nucleus (AGN) and is named after its prototype, BL Lacertae. In contrast to other types of active galactic nuclei, BL Lacs are characterized by rapid and large-amplitude flux variability and significant optical polarization.
All known BL Lacs are associated with core dominated radio sources, many of them exhibiting superluminal motion.
QSO B0323+022 is a BL Lacertae object. The image at right is taken with the ESO New Technology Telescope (NTT) using the R filter.
Relativistic jets[edit]
{{fairuse}}

{{free media}}
In the images at right are the effects of charged particles apparently moving six times the speed of light.
"We see almost a dozen clouds which appear to be moving out from the galaxy's center at between four and six times the speed of light. These are all located in a narrow [relativistic] jet of gas streaming out from the region of the black hole at the galaxy's center".[6]
"We believe this apparent speed translates into an actual velocity just slightly below that of light itself."[6]
"The speeds reported are two to three times faster than the fastest motions previously recorded in M87, the only nearby galaxy to show evidence for superluminal motion."[6]
"This discovery goes a long way towards confirming that radio galaxies, quasars and exotic BL Lac objects are basically the same beast, powered by super massive black holes, and differ only in orientation with respect to the observer".[6]
"Here we have, for the first time, a fairly normal radio galaxy with both excellent evidence for a super-massive black hole, as well as superluminal jet speeds similar to those seen in distant quasars and BL Lac objects."[6]
"This is the first time superluminal motion has been seen with any optical telescope, and this discovery was made possible by the extremely fine resolution obtained by Hubble".[7]
"The structure of relativistic jets in [active galactic nuclei] AGN on scales of light days reveals how energy propagates through jets, a process that is fundamental to galaxy evolution."[8]
Their lengths can reach several thousand[6] or even hundreds of thousands of light years.[9] The hypothesis is that the twisting of magnetic fields in the accretion disk collimates the outflow along the rotation axis of the central object, so that when conditions are suitable, a jet will emerge from each face of the accretion disk. If the jet is oriented along the line of sight to Earth, relativistic beaming will change its apparent brightness. The mechanics behind both the creation of the jets[10][11] and the composition of the jets[12] are still a matter of much debate in the scientific community; it is hypothesized that the jets are composed of an electrically neutral mixture of electrons, positrons, and protons in some proportion.
A relativistic jet emitted from the AGN of M87 is traveling at speeds between four and six times the speed of light.[6]
"The term 'superluminal motion' is something of a misnomer. While it accurately describes the speeds measured, scientists still believe the actual speed falls just below the speed of light."[6]
"It's an illusion created by the finite speed of light and rapid motion".[6]
"Our present understanding is that this 'superluminal motion' occurs when these clouds move towards Earth at speeds very close to that of light, in this case, more than 98 percent of the speed of light. At these speeds the clouds nearly keep pace with the light they emit as they move towards Earth, so when the light finally reaches us, the motion appears much more rapid than the speed of light. Since the moving clouds travel slightly slower than the speed of light, they do not actually violate Einstein's theory of relativity which sets light as the speed limit."[6]
Theoretical active galactic nuclei[edit]
{{fairuse}}
Def. a "compact region at the center of a galaxy that has a much higher than normal luminosity over at least some portion, and possibly all, of the electromagnetic spectrum"[13] is called an active galactic nucleus.
Def. a "structure formed by matter falling into a gravitational source such as a galaxy, black hole, or protostar"[14] is called an accretion disk or accretion disc.
Cosmic rays[edit]

{{free media}}
At right is an image indicating the range of cosmic-ray energies. The flux for the lowest energies (yellow zone) is mainly attributed to solar cosmic rays, intermediate energies (blue) to galactic cosmic rays, and highest energies (purple) to extragalactic cosmic rays.[15]
There is "a correlation between the arrival directions of cosmic rays with energy above 6 x 1019 electron volts and the positions of active galactic nuclei (AGN) lying within ~75 megaparsecs."[1]
Neutrons[edit]
{{fairuse}}
"Due to nγ collisions of the ultrarelativistic neutrons with the submillimeter-IR photons, the neutrons with Lorentz factors Γ > Γesc [...] should degrade in the region r ≤ rmx responsible for the low-frequency radiation of [active galactic nuclei] AGN."[16]
Gamma rays[edit]

{{free media}}
In March 2010 it was announced that active galactic nuclei are not responsible for most gamma-ray background radiation.[17] Though active galactic nuclei do produce some of the gamma-ray radiation detected here on Earth, less than 30% originates from these sources. The search now is to locate the sources for the remaining 70% or so of all gamma-rays detected. Possibilities include star forming galaxies, galactic mergers, and yet-to-be explained dark matter interactions.
Most gamma-ray emitting sources are actually gamma-ray bursts, objects which only produce gamma radiation for a few milliseconds to thousands of seconds before fading away. Only 10% of gamma-ray sources are non-transient sources. These steady gamma-ray emitters include pulsars, neutron stars, and black hole candidates such as active galactic nuclei.[18]
X-rays[edit]

{{free media}}
"[M]odels in which γ-rays are absorbed in collisions with X-rays producing nonthermal electron-positron pairs, which in turn radiate further X-rays [have been developed]."[19]
"[T]he reprocessing of radiation by e+ e- pairs could be a sufficiently robust mechanism to yield the canonical spectrum, independent of the details of the particle acceleration mechanism and the parameters of the source, such as the X- and γ-ray luminosity, L, and the size, R."[19]
"[T]he hard X-ray spectrum of a growing number of [active galactic nuclei] AGN [in] the 1-30 keV X-ray emission has four distinct components":[19]
- "an incident power law spectrum with a spectral index αix ≃ 0.9,"[19]
- "an emission line at the energy ~6.4 keV (interpreted as a fluorescent iron K-line),"[19]
- "an absorption edge at 7-8 keV (interpreted as an iron K-edge), and"[19]
- "a broad excess of emission with respect to the underlying power law at energies ≳ 10 keV (interpreted as Compton reflection from cold [T < 106 K, optically thick] material)." [19]
Super soft X-rays[edit]
Supersoft active galactic nuclei reach luminosities up to 1045 erg/s.[20]
Reds[edit]
"The combination of X-ray absorption, red near-IR continuum, polarized optical continuum, and broad lines in the majority of 2MASS [active galactic nucleus] AGNs suggests that they are viewed at an intermediate line of sight with respect to dusty, nuclear material (e.g., torus/disk/wind), as has been proposed for the similarly polarized [broad absorption line] BAL [quasi-stellar object] QSOs."[21]
Superluminals[edit]
{{fairuse}}
{{fairuse}}
{{fairuse}}
{{fairuse}}
{{fairuse}}
For active galactic nuclei (AGNs) "bright jet features typically exhibit apparent superluminal speeds and accelerated motions."[22]
AGN "jets with the fastest superluminal speeds all tend to have high Doppler boosted radio luminosities. [...] there is a correlation between intrinsic jet speed and intrinsic (de-beamed) luminosity".[22]
The AGN showing superluminal motion in B1950 are[22]
- 0003+380 S4 0003+38 J0006.1+3821 z=0.229 quasar
- 0003-066 NRAO 005 ... z=0.3467 BL Lac
- 0007+106 III Zw 2 ... z=0.0893 radio galaxy
- 0010+405 4C +40.01 ... z=0.256 quasar
- 0015-054 PMN J0017-0512 J0017.6-0510 z=0.226 quasar
- 0016+731 S5 0016+73 ··· z=1.781 quasar
- 0048-097 PKS 0048-09 J0050.6-0929 z=0.635 BL Lac
- 0055+300 NGC 315 ··· z=0.0165 radio galaxy
- 0059+581 TXS 0059+581 J0102.7+5827 z=0.644 quasar
- 0106+013 4C +01.02 J0108.6+0135 z=2.099 quasar
- 0109+224 S2 0109+22 J0112.1+2245 z=0.265 BL Lac
- 0109+351 B2 0109+35 ··· z=0.450 quasar
- 0110+318 4C +31.03 J0112.8+3208 z=0.603 quasar
- 0111+021 UGC 00773 ··· z=0.047 BL Lac
- 0116-219 OC -228 J0118.8-2142 z=1.165 quasar.
At right is a radio image of quasar S4 0003+38.[22] This object was image on March 9 and December 1, 2006, March 28 and August 24, 2007, May 1 and July 17, 2008, March 25, 2009, and July 12, 2010. From its movement as it was leaving its source in µas y-1, S4 0003+38 before it left its source was moving at 2.62±0.84c, left its source at a back projected date of 2003.01±0.24, continued accelerating to 4.63±0.32c, then began to decelerate successively at each observation epoch from 0.67±0.20, 0.36±0.32, to 0.16±0.26.[22]
The second image at right is of apparent superluminal motion in NGC 315. In both of these images the apparent motion is rectilinear or close to it. NGC 315 is a low-luminosity radio galaxy.
The third image at right shows the approximate radial motion of the jet component versus the core.
"Inward motions are rare (2% of all features), are slow (< 0.1 mas per y), are more prevalent in BL Lac jets, and are typically found within 1 mas of the unresolved core feature. [...] Considering only the AGN with a known redshift, the inward components of 1458+718 [fourth at right] are the only ones which appear significantly superluminal, ranging from 1.4 c to 4.6 c. With the exception of 1458+718, 2021+614, and 2230+114, the inward motions all occur within ∼ 1 mas of the core, in typically the innermost component. In particular, the innermost two jet components of two TeV-emitting BL Lacs in our sample: 0219+428 (3C 66A) [at fifth right] and 1219+285 (W Comae) are both inward-moving. The small velocities and core separations of these moving components may indicate that the core is not a stable reference point in these two jets."[22]
The line in the image for 1458+718 connects the two components. The "the apparent inward motion [...] of [the] two component [is] in a complex emission region located ∼ 25 mas south of the core in this compact steep spectrum quasar. [There is] one additional component in this complex [...] that is also moving inward, in a non-radial direction."[22]
"The moving features are generally non-ballistic, with 70% of the well-sampled features showing either significant accelerations or non-radial motions."[22]
"A substantial number of components showed no significant acceleration, but had non-radial motion vectors. [...] This is in stark contrast to the kinematics of features in stellar (Herbig-Haro) jets, which are well described by ballistic models [...] Of the 739 components with statistically significant (≥ 3σ) speeds, 38% exhibited significant non-radial motion, implying non-ballistic trajectories.".[22]
The "acceleration [is resolvable into] terms μ⊥ and μǁ in directions perpendicular and parallel, respectively, to the mean angular velocity direction φ."[22]
"[S]ignificant parallel accelerations [are] seen in roughly one third of our sample, and significant perpendicular accelerations in about one fifth of our sample."[22]
"VLBA images of the jet in the broad-line radio galaxy 3C 111. The picture shows the variable parsec-scale structure of the jet in this active galactic nucleus. The features observed correspond to ejected plasma regions traveling at relativistic speeds. Those appear to be larger than the speed of light due to projection effects. The sixteen images are spaced by their relative time intervals. The images show that a major radio flux-density outburst in 1996 was followed by a particularly bright plasma ejection associated with a superluminal jet component. This major event was followed by trailing features in its evolution. A similar event is seen after mid 2001. The jet dynamics in this source is revealed: a plasma injection into the jet beam leads to the formation of multiple shocks that travel at different speeds downstream (ranging from 3c to 6c) and interact with each other and with the ambient medium. This is in agreement with numerical relativistic magnetohydrodynamic structural and emission simulations of jets."[23]
"Images were taken at 15 GHz with the full Very Long Baseline Array as part of the 2cm Survey/MOJAVE collaboration. The observing runs usually last 8 hr and the total observing time on source is approximately 50 minutes. The typical dynamic range in the images is of 1000:1 (the lowest shown flux density is typically of 1-2 mJy/beam). The images are convolved with a common restoring beam of 0.5x1.0 milliarcseconds (P.A. of 0 deg). The image alignment is (arbitrary) to the brightness peak. The superluminal speeds of the features in the jet were determined from a detailed analysis of multiple Gaussian model fits to the observed visibilities."[23]
Warm–hot intergalactic medium[edit]

{{free media}}
The warm–hot intergalactic medium (WHIM) refers to a sparse, warm-to-hot (105 to 107 K) plasma that [may] exist in the spaces between galaxies and ... contain 40–50%[24] of the baryons (that is, 'normal matter' which exists as plasma or as atoms and molecules, in contrast to dark matter) in the universe at the current epoch.[25] Because of the high temperature of the medium, it is more readily observed from the ultraviolet and low energy X-ray emission. This was detected in the 0.4–0.6 keV energy band as of 2010 using the orbiting XMM-Newton observatory. This emission forms 12% ± 5% of the diffuse X-ray background radiation.[26]
Within the WHIM, gas shocks are created as a result of active galactic nuclei, along with the gravitationally-driven processes of merging and accretion. Part of the gravitational energy supplied by these effects is converted into thermal emissions of the matter by collisionless shock heating.[24]
In May 2010 a giant reservoir of WHIM was detected by the Chandra X-ray Observatory lying along the wall shaped structure of galaxies (Sculptor Wall) some 400 million light-years from Earth.[27][28]
"These computer simulations [at right] show a swarm of dark matter clumps around our Milky Way galaxy. Some of the dark-matter concentrations are massive enough to spark star formation. Thousands of clumps of dark matter coexist with our Milky Way galaxy, shown in the center of the top panel. The green blobs in the middle panel are those dark-matter chunks massive enough to obtain gas from the intergalactic medium and trigger ongoing star formation, eventually creating dwarf galaxies. In the bottom panel, the red blobs are ultra-faint dwarf galaxies that stopped forming stars long ago."[29]
Galaxies[edit]
Galaxy type | Active
nuclei |
Emission lines | X-rays | Excess of | Strong
radio |
Jets | Variable | Radio
loud | ||
---|---|---|---|---|---|---|---|---|---|---|
Narrow | Broad | UV | Far-IR | |||||||
Normal | no | weak | no | weak | no | no | no | no | no | no |
Low-ionization nuclear emission-line region (LINER) | unknown | weak | weak | weak | no | no | no | no | no | no |
Seyfert I | yes | yes | yes | some | some | yes | few | no | yes | no |
Seyfert II | yes | yes | no | some | some | yes | few | no | yes | no |
Quasar | yes | yes | yes | some | yes | yes | some | some | yes | some |
Blazar | yes | no | some | yes | yes | no | yes | yes | yes | yes |
BL Lac object | yes | no | no/faint | yes | yes | no | yes | yes | yes | yes |
OVV quasar | yes | no | stronger than BL Lac | yes | yes | no | yes | yes | yes | yes |
Radio galaxy | yes | some | some | some | some | yes | yes | yes | yes | yes |
Milky Way[edit]

{{free media}}

{{free media}}

{{free media}}
New observations from ESO’s Very Large Telescope show for the first time [in the right image] a gas cloud being ripped apart by the supermassive black hole at the centre of the galaxy. Shown here are VLT observations from 2006, 2010 and 2013, coloured blue, green and red respectively. Due to its distance, and the fact that we see the orbit at a steep angle as the cloud falls towards the black hole, only the position, not the shape, of the cloud can be discerned in this image. The stretching of the cloud is seen in observations of its velocity, which allow astronomers to work out where on its orbit the different parts of the cloud are now located.
These observations show how a gas cloud [in the left image] now passing close to the supermassive black hole at the centre of the galaxy is being ripped apart. The horizontal axis shows the extent of the cloud along its orbit and the vertical axis shows the velocities of different parts of the cloud. The cloud is now dramatically stretched out and the velocity of the front is several million km/h different from that of the tail.
These observations [center image] from ESO’s Very Large Telescope, using the SINFONI instrument, show how a gas cloud is being stretched and ripped apart as it passes close to the supermassive black hole at the centre of the galaxy. The horizontal axis shows the extent of the cloud along its orbit and the vertical axis shows the velocities of different parts of the cloud during the last ten years. The cloud is now (2013) dramatically stretched out and the velocity of the front is several million km/h different from that of the tail.
NGC 507[edit]

{{free media}}
NGC 507, also known as rp 229, CGCG 502-67, MCG 5-4-44, PGC 5098, UGC 938, and V V 207,[30] is a lenticular galaxy in the constellation Pisces. It was described as being "very faint", "pretty large", "round", "brighter in the middle", and "south of NGC 508" by John Louis Emil Dreyer in the New General Catalogue.[31] It was discovered by William Herschel on September 12, 1784.[32]
This composite image on the right shows a vast cloud of hot gas (X-ray/red), surrounding high-energy bubbles (radio/blue) on either side of the bright white area around the supermassive black hole. By studying the inner regions of the galaxy with Chandra, scientists estimated the rate at which gas is falling toward the galaxy's supermassive black hole. These data also allowed an estimate of the power required to produce the bubbles, which are each about 10,000 light years in diameter. Surprisingly, the analysis indicates that most of the energy released by the infalling gas goes into producing jets of high-energy particles that create the huge bubbles, rather than into an outpouring of light as observed in many active galactic nuclei.
NGC 1097[edit]

{{free media}}
At right is a "[c]olour-composite image of the central 5,500 light-years wide region of the spiral galaxy NGC 1097 [45 million light years away], obtained with the NACO adaptive optics on the VLT. More than 300 star forming regions - white spots in the image - are distributed along a ring of dust and gas in the image. At the centre of the ring there is a bright central source where the active galactic nucleus and its super-massive black hole are located. The image was constructed by stacking J- (blue), H- (green), and Ks-band (red) [infrared] images. North is up and East is to the left. The field of view is 24 x 29 arcsec2, i.e. less than 0.03% the size of the full moon!"[33]
NGC 1700[edit]

{{free media}}
NGC 1700 is an elliptical galaxy of the Hubble type E4 pec in the constellation Eridanus south of the ecliptic. The galaxy has an angular extent of 3.3 '× 2.1', an apparent brightness of +11.2 mag. It is about 180 million light years away from the solar system and has a diameter of about 215,000 light years.[34] It has an active galactic nucleus.
NGC 3393[edit]

{{free media}}
"I'm still on a roll with the active galactic nuclei (AGN) and here is the latest, with thanks to Mitchell Revalski et al. for the list of interesting objects to investigate. I had the usual trouble with this one trying to balance the colors while making the illuminated filaments easy to discern. In many galaxies, the details near the nucleus are not so important to convey, and it is therefore ok if it's all a bright ball. Here, the image is quite dark to accommodate the details in the core."[35]
"We're quite used to seeing spiral galaxies with uniformly yellowish cores full of old stars, so when something blue or green is spotted, it seems a bit odd, and that's one of the ways astronomers can find these fascinating galaxies. Such nuances are picked out relatively easily by comparing spectroscopic results from many different galaxies. Spectroscopy is kind of like a fingerprint in light, and whatever spikes and dips in the graph appear tell a story about how far the light traveled, what elements are present, and what's happening to those elements."[35]
"Apparently there is not just one black hole at the center of this galaxy, but a pair that are eventually going to merge. Would you believe that spectroscopy can also tell us this? This is moving into the realm of things I don't understand well enough to explain, but here are a number of papers specifically on the case of this galaxy. arxiv.org/find/all/1/all:+AND+NGC+3393/0/1/0/all/0/1"[35]
"Data from the following proposal were used to create this image: The Hosts of Megamaser Disk Galaxies"[35]
"The representation of filters was a bit difficult, as I used some near-infrared data for around the core, but it didn't extend all the way to the edge, and I had to make up for it with the F814W data there. With that in mind, colors are as follows:"[35]
Red: WFC3/IR F160W + WFC3/IR F110W Green: WFC3/UVIS F814W Blue: WFC3/UVIS F438W + WFC3/UVIS F336W
"North is NOT up. It is 38.67° counter-clockwise from up."[35]
NGC 3621[edit]

{{free media}}
This image on the right, from ESO’s Very Large Telescope (VLT), shows a truly remarkable galaxy known as NGC 3621. To begin with, it is a pure-disc galaxy. Like other spirals, it has a flat disc permeated by dark lanes of material and with prominent spiral arms where young stars are forming in clusters (the blue dots seen in the image). But while most spiral galaxies have a central bulge — a large group of old stars packed in a compact, spheroidal region — NGC 3621 doesn’t. In this image, it is clear that there is simply a brightening to the centre, but no actual bulge like the one in NGC 6744 (eso1118), for example.
NGC 3621 is also interesting as it is believed to have an active supermassive black hole at its centre that is engulfing matter and producing radiation. This is somewhat unusual because most of these so-called active galactic nuclei exist in galaxies with prominent bulges. In this particular case, the supermassive black hole is thought to have a relatively small mass, of around 20 000 times that of the Sun.
Another interesting feature is that there are also thought to be two smaller black holes, with masses of a few thousand times that of the Sun, near the nucleus of the galaxy. Therefore, NGC 3621 is an extremely interesting object which, despite not having a central bulge, has a system of three black holes in its central region.
This galaxy is located in the constellation of Hydra (The Sea Snake) and can be seen with a moderate-sized telescope. This image, taken using B, V, and I filters with the FORS1 instrument on the powerful VLT, shows striking detail in this odd object and also reveals a multitude of background galaxies. A number of bright foreground stars that belong to our own Milky Way are also visible.
NGC 3945[edit]

{{free media}}
Image on the right is just a cool looking lenticular galaxy in the archive. It was shaped something like a TIE fighter but it turns out the ring goes all the way around. This is only the central part. Another ring of matter encircles the galaxy further out of the frame. Here is a complete image and interesting accompanying arguments about the actual shape of the galaxy. Can you tell just from looking at it what its three dimensional shape is? Is it a oblique disk, an oblate sphere, or a combination of the two?
Since this is an infrared image, the spiral structures visible in image at the link in the above paragraph are not visible in this image.
Data is from proposal StSci. ID 11219: Active Galactic Nuclei in nearby galaxies: a new view of the origin of the radio-loud radio-quiet dichotomy?
North is NOT up. It is 14.6° counter-clockwise from up.
NGC 4321[edit]

{{free media}}
"This [visual] image [at right] from the NASA/ESA Hubble Space Telescope, the most detailed made to date, shows the bright core of the galaxy and the innermost parts of its spiral arms. Messier 100 has an active galactic nucleus — a bright region at the galaxy’s core caused by a supermassive black hole that is actively swallowing material, which radiates brightly as it falls inwards."[36] Bold added.
"Messier 100 is a perfect example of a grand design spiral galaxy, a type of galaxy with prominent and very well-defined spiral arms. These dusty structures swirl around the galaxy’s nucleus, and are marked by a flurry of star formation activity that dots Messier 100 with bright blue, high-mass stars."[36]
"The galaxy’s spiral arms also host smaller black holes, including the youngest ever observed in our cosmic neighbourhood, the result of a supernova observed in 1979."[36]
"Messier 100 is located in the direction of the constellation of Coma Berenices, about 50 million light-years distant."[36]
"This image, taken with the high resolution channel of Hubble’s Advanced Camera for Surveys demonstrates the continued evolution of Hubble’s capabilities over two decades in orbit. This image, like all high resolution channel images, has a relatively small field of view: only around 25 by 25 arcseconds."[36]
The visual data is centered at 555 nm (blue), the visual + infrared is in green, and additional infrared centered at 814 nm is red.[36]
NGC 4435[edit]

{{free media}}
NGC 4435 is a barred lenticular galaxy currently interacting with NGC 4438. Studies of the galaxy by the Spitzer Space Telescope revealed a relatively young (190 million years) stellar population within the galaxy's nucleus, which may have originated through the interaction with NGC 4438 compressing gas and dust in that region, triggering a starburst.[37] It also has a long tidal tail possibly caused by the interaction with the mentioned galaxy;[38] however, other studies suggest that tail is actually a galactic cirrus in the Milky Way totally unrelated to NGC 4435.[39]
NGC 5548[edit]

{{free media}}
NGC 5548 is a Type I Seyfert galaxy with a bright blue/white core. NGC 5548 is approximately 245[40] million light years away and appears in the constellation Boötes. This galaxy was studied by the Multicolor Active Galactic Nuclei Monitoring 2 m telescope.[41]
By the galaxy morphological classification, this is an unbarred lenticular galaxy with tightly-wound spiral arms, while shell and tidal tail features suggest that it has undergone a cosmologically-recent merger or interaction event.[42]
Observation of NGC 5548 during the 1960s with radio telescopes showed an enhanced level of radio emission.[43] Spectrograms of the nucleus made in 1966 showed that the energized region was confined to a volume a few parsecs across, where temperature were around 14000 K and the plasma had a dispersion velocity of ±450 km/s.[44]
There is "a clumpy gas stream flowing quickly outwards and blocking 90 percent of the X-rays emitted by the black hole. This activity could provide insights into how supermassive black holes interact with their host galaxies."[45]
"The discovery of the unusual behaviour in NGC 5548 is the result of an intensive observing campaign using major ESA and NASA space observatories: ESA’s X-ray Multi-Mirror Mission (XMM-Newton), the NASA/ESA Hubble Space Telescope, NASA’s Swift, NASA’s Nuclear Spectroscopic Telescope Array (NuSTAR), NASA’s Chandra X-ray Observatory, and ESA's International Gamma-Ray Astrophysics Laboratory (INTEGRAL)."[45]
"An active galaxy is a galaxy which hosts an active galactic nucleus (AGN). An AGN is a compact region at the centre of a galaxy that has a much higher than normal luminosity. The high level of radiation, sometimes across the whole of the electromagnetic spectrum, is thought to be a result the supermassive black hole at the centre pulling in mass from the surroundings."[45]
"There are other galaxies that show gas streams near a black hole, but this is the first time that a stream like this has been seen to move into the line of sight."[45]
"This is the first direct evidence for the long-predicted shielding process that is needed to accelerate powerful gas streams, or winds, to high speeds."[46]
"We were very lucky. You don’t normally see this kind of event with objects like this. It tells us more about the powerful ionised winds that allow supermassive black holes in the nuclei of active galaxies to expel large amounts of matter. In larger quasars than NGC 5548, these winds can regulate the growth of both the black hole and its host galaxy."[46]
"As matter spirals down into a black hole it forms a flat disc, known as an accretion disc. The disc is heated so much that it emits X-rays, near to the black hole, and less energetic ultraviolet radiation further out. The ultraviolet radiation can create winds strong enough to blow gas away from the black hole, which otherwise would have fallen into it. But, the winds only come into existence if their starting point is shielded from X-rays."[45]
"Earlier observations had seen the effects of both X-rays and ultraviolet radiation on a region of warm gas for away from the black hole, but these most recent observations have shown the presence of a new gas stream between the disc and the original cloud. The newly discovered gas stream in the archetypal Seyfert galaxy NGC 5548 — one of the best-studied sources of this type over the past half-century — absorbs most of the X-ray radiation before it reaches the original cloud, shielding it from X-rays and leaving only the ultraviolet radiation. The same stream shields gas closer to the accretion disc. This makes the strong winds possible, and it appears that the shielding has been going on for at least three years."[45]
“There were dramatic changes since the last observation with Hubble in 2011. We saw signatures of much colder gas than was present before, indicating that the wind had cooled down, due to a strong decrease in the ionising X-ray radiation from the nucleus."[47]
"NGC 5548’s persistent wind, which has been known about for two decades, reaches velocities exceeding 3.5 million kilometres per hour. But, a new wind has arisen which is much stronger and faster than the persistent wind."[45]
"The new wind reaches speeds of up to 18 million kilometres per hour, but is much closer to the nucleus than the persistent wind. The new gas outflow blocks 90 percent of the low-energy X-rays that come from very close to the black hole, and it obscures up to a third of the region that emits the ultraviolet radiation at a distance of a few light-days from the black hole."[46]
"Strong X-ray absorption by ionised gas has been seen in several other sources, and it has been attributed for instance to passing clouds."[45]
"However, in our case, thanks to the combined XMM-Newton and Hubble data, we know this is a fast stream of outflowing gas very close to the nucleus"[48]
"It may even originate from the accretion disc."[49]
UGC 6093[edit]

{{free media}}
UGC 6093 is classified as an active galaxy, which means that it hosts an active galactic nucleus.[50]
"This image, captured by the NASA/ESA Hubble Space Telescope’s Wide Field Camera 3 (WFC3), shows a galaxy named UGC 6093. As can be easily seen, UGC 6093 is something known as a barred spiral galaxy — it has beautiful arms that swirl outwards from a bar slicing through the galaxy’s centre. It is classified as an active galaxy, which means that it hosts an active galactic nucleus, or AGN: a compact region at a galaxy’s centre within which material is dragged towards a supermassive black hole. As this black hole devours the surrounding matter it emits intense radiation, causing it to shine brightly."[50]
"But UGC 6093 is more exotic still. The galaxy essentially acts as a giant astronomical laser that spews out light at microwave, not visible, wavelengths — this type of object is dubbed a megamaser (maser being the term for a microwave laser). Megamasers such as UGC 6093 can be some 100 million times brighter than masers found in galaxies like the Milky Way."[50]
"Hubble’s WFC3 observes light spanning a range wavelengths — from the near-infrared, through the visible range, to the near-ultraviolet. It has two channels that detect and process different light, allowing astronomers to study a remarkable range of astrophysical phenomena; for example, the UV-visible channel can study galaxies undergoing massive star formation, while the near-infrared channel can study redshifted light from galaxies in the distant Universe. Such multi-band imaging makes Hubble invaluable in studying megamaser galaxies, as it is able to untangle their intriguing complexity."[50]
IC 4970[edit]

{{free media}}
"This composite image of data from three different telescopes shows an ongoing collision between two galaxies, NGC 6872 and IC 4970. X-ray data from NASA's Chandra X-ray Observatory is shown in purple, while Spitzer Space Telescope's infrared data is red and optical data from ESO's Very Large Telescope (VLT) is colored red, green and blue."[51]
"Astronomers think that supermassive black holes exist at the center of most galaxies. Not only do the galaxies and black holes seem to co-exist, they are apparently inextricably linked in their evolution. To better understand this symbiotic relationship, scientists have turned to rapidly growing black holes -- so-called active galactic nucleus (AGN) -- to study how they are affected by their galactic environments."[51]
"The latest data from Chandra and Spitzer show that IC 4970, the small galaxy at the top of the image, contains an AGN, but one that is heavily cocooned in gas and dust. This means in optical light telescopes, like the VLT, there is little to see. X-rays and infrared light, however, can penetrate this veil of material and reveal the light show that is generated as material heats up before falling onto the black hole (seen as a bright point-like source)."[51]
"Despite this obscuring gas and dust around IC 4970, the Chandra data suggest that there is not enough hot gas in IC 4970 to fuel the growth of the AGN. Where, then, does the food supply for this black hole come from? The answer lies with its partner galaxy, NGC 6872. These two galaxies are in the process of undergoing a collision, and the gravitational attraction from IC 4970 has likely pulled over some of NGC 6872's deep reservoir of cold gas (seen prominently in the Spitzer data), providing a new fuel supply to power the giant black hole."[51]
3C 279[edit]

{{free media}}

{{free media}}
3C 279 (also known as 4C–05.55, NRAO 413, and PKS 1253–05) is an optically violent variable quasar (OVV), which exhibits variations in the visible, radio, and X-ray bands.[52] The quasar was observed to have undergone a period of extreme activity from 1987 until 1991.[53] The Rosemary Hill Observatory (RHO) started observing 3C 279 in 1971,[53] the object was further observed by the Compton Gamma Ray Observatory in 1991, when it was unexpectedly discovered to be one of the brightest gamma ray objects in the sky.[54] It is also one of the brightest and most variable sources in the gamma ray sky monitored by the Fermi Space Telescope.
Apparent superluminal motion was detected during observations first made in 1973 in a jet of material departing from the quasar.[55]
In the second image down on the right, (a) is validation of Continuous High-Resolution Image Reconstruction Using Patch Priors (CHIRP) on data from the Boston University Blazar Group demonstrating superresolution [46,47] and (b) is a comparison of CHIRP (trained on natural images) and BiSpectrum Maximum Entropy Method (BSMEM) on reconstructing a black hole model image (courtesy A. Broderick) from simulated data.[56]
MCG+01-38-005[edit]

{{free media}}
"Phenomena across the universe emit radiation spanning the entire electromagnetic spectrum — from high-energy gamma rays, which stream out from the most energetic events in the cosmos, to lower-energy microwaves and radio waves."[57]
"Microwaves, the very same radiation that can heat up your dinner, are produced by a multitude of astrophysical sources, including strong emitters known as masers (microwave lasers), even stronger emitters with the somewhat villainous name of megamasers, and the centers of some galaxies. Especially intense and luminous galactic centers are known as active galactic nuclei. They are in turn thought to be driven by the presence of supermassive black holes, which drag surrounding material inwards and spit out bright jets and radiation as they do so."[57]
"The two galaxies shown here, imaged by the Hubble Space Telescope, are named MCG+01-38-004 (the upper, red-tinted one) and MCG+01-38-005 (the lower, blue-tinted one). MCG+01-38-005 is a special kind of megamaser; the galaxy’s active galactic nucleus pumps out huge amounts of energy, which stimulates clouds of surrounding water. Water’s constituent atoms of hydrogen and oxygen are able to absorb some of this energy and re-emit it at specific wavelengths, one of which falls within the microwave regime. MCG+01-38-005 is thus known as a water megamaser!"[57]
"Astronomers can use such objects to probe the fundamental properties of the universe. The microwave emissions from MCG+01-38-005 were used to calculate a refined value for the Hubble constant, a measure of how fast the universe is expanding. This constant is named after the astronomer whose observations were responsible for the discovery of the expanding universe and after whom the Hubble Space Telescope was named, Edwin Hubble."[57]
Circinus galaxy[edit]
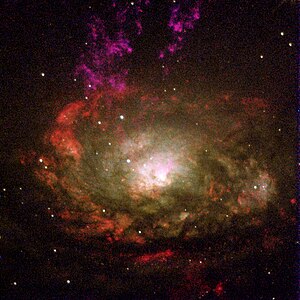
{{free media}}
"Resembling a swirling witch's cauldron of glowing vapors, the black hole-powered core of a nearby active galaxy appears in this colorful NASA Hubble Space Telescope image. The galaxy lies 13 million light-years away in the southern constellation Circinus."[58]
"This galaxy is designated a type 2 Seyfert, a class of mostly spiral galaxies that have compact centers and are believed to contain massive black holes. Seyfert galaxies are themselves part of a larger class of objects called Active Galactic Nuclei or AGN. AGN have the ability to remove gas from the centers of their galaxies by blowing it out into space at phenomenal speeds. Astronomers studying the Circinus galaxy are seeing evidence of a powerful AGN at the center of this galaxy as well."[58]
"Much of the gas in the disk of the Circinus spiral is concentrated in two specific rings - a larger one of diameter 1,300 light-years, which has already been observed by ground-based telescopes, and a previously unseen ring of diameter 260 light-years."[58]
"In the Hubble image, the smaller inner ring is located on the inside of the green disk. The larger outer ring extends off the image and is in the plane of the galaxy's disk. Both rings are home to large amounts of gas and dust as well as areas of major "starburst" activity, where new stars are rapidly forming on timescales of 40 - 150 million years, much shorter than the age of the entire galaxy."[58]
"At the center of the starburst rings is the Seyfert nucleus, the believed signature of a supermassive black hole that is accreting surrounding gas and dust. The black hole and its accretion disk are expelling gas out of the galaxy's disk and into its halo (the region above and below the disk). The detailed structure of this gas is seen as magenta-colored streamers extending towards the top of the image."[58]
"In the center of the galaxy and within the inner starburst ring is a V-shaped structure of gas. The structure appears whitish-pink in this composite image, made up of four filters. Two filters capture the narrow lines from atomic transitions in oxygen and hydrogen; two wider filters detect green and near-infrared light. In the narrow-band filters, the V-shaped structure is very pronounced. This region, which is the projection of a three-dimensional cone extending from the nucleus to the galaxy's halo, contains gas that has been heated by radiation emitted by the accreting black hole. A "counter-cone," believed to be present, is obscured from view by dust in the galaxy's disk. Ultraviolet radiation emerging from the central source excites nearby gas causing it to glow. The excited gas is beamed into the oppositely directed cones like two giant searchlights."[58]
"Located near the plane of our own Milky Way Galaxy, the Circinus galaxy is partially hidden by intervening dust along our line of sight. As a result, the galaxy went unnoticed until about 25 years ago. This Hubble image was taken on April 10, 1999 with the Wide Field Planetary Camera 2."[58]
Hickson Compact Group 59[edit]

{{free media}}
"That galaxies come in very different shapes and sizes is dramatically demonstrated by this striking Hubble image of the Hickson Compact Group 59. Named by astronomer Paul Hickson in 1982, this is the 59th such collection of galaxies in his catalogue of unusually close groups. What makes this image interesting is the variety on display. There are two large spiral galaxies, one face-on with smooth arms and delicate dust tendrils, and one highly inclined, as well as a strangely disorderly galaxy featuring clumps of blue young stars. We can also see many apparently smaller, probably more distant, galaxies visible in the background. Hickson groups display many peculiarities, often emitting in the radio and infrared and featuring active star-forming regions. In addition their galaxies frequently contain Active Galactic Nuclei powered by supermassive black holes, as well large quantities of dark matter."[59]
"The NASA/ESA Hubble Space Telescope's Advanced Camera for Surveys, using the Wide Field Channel, captured this image of HCG059 in 2007. The picture was created from images taken through blue, yellow and near-infrared filters (F435W, F606W and F814W). The total exposure times per filter were 57 minutes, 41 minutes and 35 minutes respectively. The field of view is about 3.4 arcminutes across."[59]
Galactic mergers[edit]

{{free media}}
"A team of astronomers studied over 1400 galaxies snapped by Hubble in the COSMOS survey, to test the hypothesis that galactic mergers trigger active galactic nuclei (AGN). In order to make this a blind test, the team modelled and removed the active nucleus (which normally appears as a bright spot) from each galaxy, and then cosmetically added a similar mark to the galaxies without an AGN, to make them visually indistinguishable. This explains the black dot visible near the centre of each of these images."[60]
"They then categorised the galaxies, according to whether they showed no sign of recent mergers (top), minor signs (middle row) or clear signs of disruption from a recent merger (bottom)."[60]
"Analysing these results, they found that galaxies with active nuclei (left) and those with inactive nuclei (right) showed no statistically significant difference in the proportion that had undergone mergers. This means that processes other than galactic mergers must trigger AGN activity."[60]
The Cosmic Evolution Survey (COSMOS) is a Hubble Space Telescope (HST) Treasury Project to survey a two square degree equatorial field with the Advanced Camera for Surveys (ACS).[61] The largest survey ever undertaken by HST, the project incorporates commitments from observatories around the world, such as the Very Large Array radio observatory, the European Space Agency's XMM-Newton satellite, and Japan's eight meter Subaru telescope. At the moment, more than 150 astronomers around the world actively contribute to the project.
The project's primary goal is to study the relationship between large scale structure (LSS) in the universe and dark matter, the formation of galaxies, and nuclear activity in galaxies. This includes careful analysis of the dependence of galaxy evolution on environment.[62] The survey covers 2 square degrees of sky in the constellation Sextans.
SDSSJ0150+2725[edit]

{{free media}}
"Though the bright, light-speckled foreground galaxy on the left is eye-catching, it is far from the most intriguing object in this NASA/ESA Hubble Space Telescope image. In the upper part of the frame, the light from distant galaxies has been smeared and twisted into odd shapes, arcs, and streaks. This phenomenon indicates the presence of a giant galaxy cluster, which is bending the light coming from the galaxies behind it with its monstrous gravitational influence."[63]
"This cluster, called SDSSJ0150+2725, lies some three billion light-years away and was first documented by the Sloan Digital Sky Survey (SDSS), hence its name. The SDSS uses a 2.5-metre optical telescope located at the Apache Point Observatory in New Mexico to observe millions of objects and create detailed 3D maps of the Universe. This particular cluster was part of the Sloan Giant Arcs Survey (SGAS), which detected galaxy clusters with strong lensing properties; their gravity stretches and warps the light of more distant galaxies sitting behind them, creating weird and spectacular arcs such as those seen here."[63]
"The Hubble data on of SDSSJ0150+2725 were part of a study of star formation in brightest cluster galaxies (called BCGs), lying between approximately 2 and 6 billion light-years away. This study found the star formation rate in these galaxies to be low, which is consistent with models that suggest that most stars in such galaxies form very early on. These BCGs also emit strong radio signals thought to be from active galactic nuclei (AGN) at their centers, suggesting that the activity from both the AGN and any ongoing star formation is fuelled by cold gas found within the host galaxies."[63]
SDSS J1336-0331[edit]

{{free media}}
"At first glance, it may seem as though this image was taken through a faulty lens, but the mind-bending distortions visible in this Hubble Wide Field Camera 3 impressive image are actually caused by a cosmic phenomenon."[64]
"The bright object at the centre of the frame is the galaxy cluster SDSS J1336-0331. The enormous gravitational influence of the cluster warps the very shape and fabric of its environment (the spacetime around it) creating an effect known as strong gravitational lensing. Through this the light from background galaxies in the line of sight to the observer are bent into fantastic arcs. This effect is very useful for studying distant background galaxies."[64]
"Moreover SDSS J1336-0331 is interesting in itself: the cluster was part of a study of star formation within 42 of the Brightest Cluster Galaxies (BCGs — the brightest galaxies within their host clusters, as the name would suggest). Typically located in the centres of their clusters, BCGs are among the most massive and luminous galaxies in the Universe. They are generally huge elliptical galaxies and are likely to host active galactic nuclei (AGN) in their cores. The study found evidence to suggest that BCGs are fueled by cold gas from the galaxy. It also showed that star formation in older BCGs no longer significantly contributes to the galaxy’s growth; instead, the stellar growth occurs through mergers, the collision of two galaxies. Violent, gas-rich major mergers can trigger intense bursts of star formation in their aftermath."[64]
SDSS J1354+1327[edit]

{{free media}}
"Researchers using a suite of telescopes including the NASA/ESA Hubble Space Telescope have spotted a supermassive black hole blowing huge bubbles of hot, bright gas — one bubble is currently expanding outwards from the black hole, while another older bubble slowly fades away. This cosmic behemoth sits within the galaxy at the bottom of this image, which lies 900 million light-years from Earth and is known as SDSS J1354+1327. The upper, larger, galaxy is known as SDSS J1354+1328."[65]
"Supermassive which can have a mass equivalent to billions of suns, are found in the centre of most galaxies (including the Milky Way). These black holes are able to “feed” on their surroundings, causing them to shine brilliantly as Active Galactic Nuclei (AGN). However, this feeding process is not continuous as it depends on how much matter is available for the black hole to consume; if the surrounding material is clumpy and irregular, an AGN can be seen turning “off” and “on”, and flickering over long cosmic timescales."[65]
"This clumpy accretion is what scientists believe has happened with the black hole in SDSS J1354+1327. Scientists believe these two outflows of material are the result of the black hole burping out material after two different feeding events. The first outburst created the fading southern relic: a cone of gas measuring 33 000 light-years across. Around 100 000 years later, a second burst spawned the more compact and radiant outflow emanating from the top of the galaxy: a cone of shocked gas some 3300 light-years across."[65]
Great Observatories Origins Deep Survey[edit]

{{free media}}
"More than 12 billion years of cosmic history are shown in this unprecedented, panoramic, full-color view of thousands of galaxies in various stages of assembly."[66]
"This image, taken by NASA's Hubble Space Telescope, was made from mosaics taken in September and October 2009 with the newly installed Wide Field Camera 3 (WFC3) and in 2004 with the Advanced Camera for Surveys (ACS). The view covers a portion of the southern field of a large galaxy census called the Great Observatories Origins Deep Survey (GOODS), a deep-sky study by several observatories to trace the formation and evolution of galaxies."[66]
"The final image combines a broad range of colors, from the ultraviolet, through visible light, and into the near-infrared. Such a detailed multi-color view of the universe has never before been assembled in such a combination of color, clarity, accuracy, and depth."[66]
"Hubble's sharp resolution and new color versatility, produced by combining data from the two cameras, are allowing astronomers to sort out the various stages of galaxy formation. The image reveals galaxy shapes that appear increasingly chaotic at each earlier epoch, as galaxies grew through accretion, collisions, and mergers. The galaxies range from the mature spirals and ellipticals in the foreground, to smaller, fainter, irregularly shaped galaxies, most of which are farther away, and therefore existed farther back in time. These smaller galaxies are considered the building blocks of the larger galaxies we see today."[66]
"Astronomers are using this multi-color panorama to trace many details of galaxy assembly over cosmic time, including the star-formation rate in galaxies, the rate of mergers among galaxies, and the abundance of weak active galactic nuclei."[66]
"The image shows a rich tapestry of 7,500 galaxies stretching back through most of the universe's history. The closest galaxies seen in the foreground emitted their observed light about a billion years ago. The farthest galaxies, a few of the very faint red specks, are seen as they appeared more than 13 billion years ago, or roughly 650 million years after the Big Bang. This mosaic spans a slice of space that is equal to about a third of the diameter of the full Moon (10 arcminutes)."[66]
"The new Hubble view highlights a wide variety of stages in the galaxy assembly process. Ultraviolet light taken by WFC3 shows the blue glow of hot, young stars in galaxies teeming with star birth. The orange light reveals the final buildup of massive galaxies about 8 billion to 10 billion years ago. The near-infrared light displays the red glow of very distant galaxies – in a few cases as far as 12 billion to 13 billion light-years away – whose light has been stretched, like a toy Slinky, from ultraviolet light to longer-wavelength infrared light due to the expansion of the universe."[66]
"In this ambitious use of Hubble's observing time, astronomers used 96 Hubble orbits to make the ACS optical observations of this slice of the GOODS field and 104 orbits to make the WFC3 ultraviolet and near-infrared exposures. WFC3 peered deeper into the universe in this study than comparable near-infrared observations from ground-based telescopes. This set of unique new Hubble observations reveals galaxies to about 27th magnitude in brightness over a factor of 10 in wavelength. That's over 250 million times fainter than the unaided eye can see in visual light from a dark ground-based site."[66]
Wisconsinian glacial[edit]
Wisconsinian glacial began at 80,000 yr BP.[67]
The "emission phenomena observed in active galactic nuclei [includes] the production of compact radio sources separating at superluminal speeds".[68]
Outbursts "of cosmic ray electrons from the Galactic Center [may] penetrate the Galaxy relatively undamped and [may be able] to have a major impact on the Solar System through their ability to vaporize and inject cometary material into the interplanetary environment. [One] such 'superwave', passing through the Solar System toward the end of the Last Ice Age, [may have been] responsible for producing major changes in the Earth's climate and for indirectly precipitating the terminal Pleistocene extinction episode. The high concentration of 10Be, NO3-, Ir and Ni observed in Late Wisconsin polar ice are consistent with this scenario."[68]
Technology[edit]

{{free media}}

{{free media}}

{{free media}}

{{free media}}

{{free media}}

{{free media}}
Validation and potential of polarimetric MEM imaging [42] is shown in the image at right. (a) Full-polarimetric images of 3C 279 from 7 mm VLBA data using CLEAN and MEM are nearly identical when the MEM image is convolved with the CLEAN beam. (b) Compared against a model image of Sgr A* at 1.3 mm, the normalized root-mean-square error of a polarimetric MEM reconstruction achieves its minimum at finer angular resolution than does CLEAN. MEM and CLEAN images reconstructed at the optimum beam sizes for each technique and the nominal beam size demonstrate that polarimetric MEM achieves superior resolution and image fidelity.[56]
In the image on the left for the Event Horizon Telescope (EHT) is 1.3 mm baseline coverage for a source at +40◦ declination. Baselines to ALMA are shown in red. ALMA baselines extend north-south coverage and are especially important for southern sources, where they fill in a large gap in the (u, v) plane between the intra–northern hemisphere baselines and the very long baselines to the South Pole Telescope.[56]
In the center image is a BSSpM reconstruction of a disk-jet model of M87 at 1.3 mm: (a) The original model [45], (b) The model convolved with a 10 μas Gaussian, (c) The BSSpM reconstruction of the image from simulated data [44], where the fringe spacing of the longest baseline is approximately 25 μas, and (d) The BSSpM reconstruction convolved with a 10 μas Gaussian, where BSSpM successfully recovers features of the model at a superresolution of 40% of the fringe spacing.[56]
In the second image down on the right, (a) shows validation of CHIRP on data from the Boston University Blazar Group demonstrating superresolution [46,47]. (b) is a comparison of CHIRP (trained on natural images) and BSMEM on reconstructing a black hole model image (courtesy A. Broderick) from simulated data. CHIRP is more robust as the signal-to-noise ratio of the simulated data is lowered.[56]
For the second image down on the left, "EHT 1.3 mm baseline coverage for a source at −20◦. Baselines to ALMA are shown in red. ALMA baselines extend north-south coverage and are especially important for southern sources, where they fill in a large gap in the (u, v) plane between the intra–northern hemisphere baselines and the very long baselines to the South Pole Telescope."[56]
For the second centered image down, "(a) Full-polarimetric images of 3C 279 from 7 mm VLBA data using CLEAN and MEM are nearly identical when the MEM image is convolved with the CLEAN beam. (b) Compared against a model image of Sgr A* at 1.3 mm, the normalized root-mean-square error of a polarimetric MEM reconstruction achieves its minimum at finer angular resolution than does CLEAN. MEM and CLEAN images reconstructed at the optimum beam sizes for each technique and the nominal beam size demonstrate that polarimetric MEM achieves superior resolution and image fidelity."[56]
Image stacking[edit]

{{free media}}
"These two images show "stacked" Chandra images for two different classes of distant, massive galaxy detected with Spitzer. Image stacking is a procedure used to detect emission from objects that is too faint to be detected in single images. To enhance the signal, images of these faint objects are stacked on top of one another."[69]
"In both images, low-energy X-rays are shown in orange and high-energy X-rays in blue, and the stacked object is in the center of the image (the other sources beyond the center of the image are X-ray sources that were directly detected and are not part of the source stacking)."[69]
"On the left is a stacked Chandra image of the "normal" galaxies seen with Spitzer. The infrared emission for these young, massive galaxies is consistent with expectations for star formation. The Chandra image shows mainly low-energy X-ray emission at the center as expected."[69]
"On the right, is a stacked Chandra image for galaxies with infrared emission exceeding the levels likely to be caused by star formation. These galaxies contain active galactic nuclei, or quasars, in their centers. These are luminous objects powered by the rapid growth of supermassive black holes. The obscured quasars show much higher levels of high-energy X-ray emission because the less energetic X-rays are mostly absorbed by gas."[69]
EXOSAT[edit]

{{free media}}

{{free media}}
The European X-ray Observatory Satellite (EXOSAT), originally named HELOS, was an X-ray telescope operational from May 1983 until April 1986 and in that time made 1780 observations in the X-ray band of most classes of astronomical object including active galactic nuclei, stellar coronae, cataclysmic variables, white dwarfs, X-ray binaries, clusters of galaxies, and supernova remnants.
This European Space Agency (ESA) satellite for direct-pointing and lunar-occultation observation of X-ray sources beyond the solar system was launched into a highly eccentric orbit (apogee 200,000 km, perigee 500 km) almost perpendicular to that of the moon on May 26, 1983. The instrumentation includes two low-energy imaging telescopes (LEIT) with Wolter I X-ray optics (for the 0.04-2 keV energy range), a medium-energy experiment using Ar/CO2 and Xe/CO2 detectors (for 1.5-50 keV), a Xe/He gas scintillation spectrometer (GSPC) (covering 2-80 keV), and a reprogrammable onboard data-processing computer. Exosat was capable of observing an object (in the direct-pointing mode) for up to 80 hours and of locating sources to within at least 10 arcsec with the LEIT and about 2 arcsec with GSPC.[70]
"Thirty-four years ago, on 26 May 1983, ESA’s Exosat satellite was launched by a Thor-Delta rocket from Vandenburg Airforce Base, California, USA, and was taken over by mission controllers at ESOC, the European Space Operations Centre, Darmstadt, Germany."[71]
"Designed to observe and detect high-energy sources, Exosat was the first ESA mission to study the Universe at X-ray wavelengths, and one of the first uncrewed satellites to feature an on-board computer."[71]
"By placing the satellite in an elliptical orbit, mission teams were able to operate the instruments for 76 hours of each revolution."[71]
"In its three-year life, the mission observed a wide variety of objects, including active galactic nuclei, X-ray binary systems, supernova remnants and clusters of galaxies."[71]
"The results that Exosat obtained were very useful to scientists, and led to several new discoveries. The most important of these was probably the discovery of quasi-periodic oscillations in low-mass X-ray binary stars and X-ray pulsars, a phenomenon unknown before Exosat. All the data that Exosat retrieved are still available for study, and are still leading to new discoveries."[71]
Simeiz RT-22 telescope[edit]

{{free media}}
"22-m radio telescope for mm and cm radio waves. Located at the foot of mount Koshka (Cat) in Katsiveli (near Simeiz). Belongs to the Crimean Astrophysical Observatory, the Department of Radioastronomy."[72]
"RT-22 operation is supported by control system, consisting of two encoders with accuracy about 3 arcsec (rms), personal computer, CAMAC, quartz time standard, electric engines and other needed equipment and software. The fast RT-22 movement is supported by 2 engines with 20 kW power each, they are used for rapid change of the pointing or to move RT-22 from one source to another. 2 kW engines are used for tracking of the source."[72]
"Diameter: 22 m. Surface tolerance: 0.25 mm. Wavelength limit: 2 mm."[72]
"The radiotelescope is able to observe only single circular polarization which can be selected by investigator. Radiotelescope has a feed horn which allows to observe at the wavelength 13 and 3.6 cm simultaneously. The radiotelescope operation is supported by a control system, which consists of two encoders with accuracy of positioning 3 arcsec (rms), computer IBM-486, CAMAC, quartz time standard, electric engines and other equipment and software. 2 engines with 20 kW power each provide the fast radiotelescope motion and they are used for rapid change of the pointing or for radiotelescope motion from one source to another. Another two 2 kW engines are used for slow tracking of the source."[72]
"The control system of the radiotelescope provides the possibility to point the antenna and to track observed source in two regimes: autonomous and automatic. Operator sets the coordinates of the targeted source using keyboard of the computer when the system works in autonomous regime. All modes of the radiotelescope operation: antenna motion, radiometer readings, data recording are given from the special host computer in automatic regime."[72]
"The radiotelescope doesn't have a cover sheet (radome). Low frequencies equipment and recording system are located in the laboratory building in 30 meters apart from the radiotelescope."[72]
"The Department provides observing facilities for astronomers of international community and for its own staff. The following projects currently run:"[72]
- Very Long Baseline Interferometry (VLBI).
- Multi-wavelength monitoring of Active Galactic Nuclei (AGN).
- Solar and stellar activity investigations.
- Molecular lines observations at mm wavelengths.
Telescopes at La Silla[edit]

{{free media}}
In this photograph, three telescopes are portrayed, all looking very different from each other. To the right of the water tanks is the ESO New Technology Telescope (NTT), which had its first light on 23 March 1989. This 3.58-metre telescope was the first ever to have a computer-controlled main mirror, which could adjust its shape during observations to optimise image quality. The octagonal enclosure housing the NTT is another technological breakthrough, ventilated by a system of flaps that makes air flow smoothly across the mirror, reducing turbulence and leading to sharper images.
To the right of the NTT is the Swiss 1.2-metre Leonhard Euler Telescope, which has a more traditional dome-shaped enclosure. It is operated by the Geneva Observatory at the Université de Genève in Switzerland, and had its first light on 12 April 1998. It is used to search for exoplanets in the southern sky; with its first discovery being a planet in orbit around the star Gliese 86. The telescope also observes variable stars, gamma-ray bursts and active galactic nuclei.
In the foreground on the right is a building nicknamed the sarcofago (sarcophagus). This houses the TAROT (Télescope à Action Rapide pour les Objets Transitoires, or Rapid Action Telescope for Transient Objects), which started work at La Silla on 15 September 2006.
Tuorla Observatory[edit]
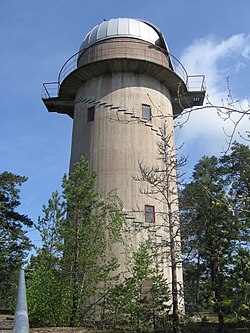
{{free media}}
Tuorla is located about 12 kilometres from Turku in the direction of Helsinki. The observatory is at an altitude of 60.6 m above sea level (asl).
A new observatory was needed because the old Iso-Heikkilä Observatory close to the centre of Turku started suffering heavy light pollution from nearby city and especially industrial areas to the south of the observatory. A new place was found in Tuorla, which is one of the small villages in (former) Piikkiö municipality.
The observatory has several telescopes located around the main buildings and also international telescopes like the Nordic Optical Telescope are in use. The one meter Dall-Kirkham reflector ([1]) is the largest optical telescope in Finland.
The main area of research in Tuorla is active galactic nuclei; about half of the researchers are working on the topic. Other areas are dark matter, cosmology, astrodynamics, binary stars, solar neighborhood, solar physics and astrobiology. The optical laboratory produces high quality optics for telescopes.
See also[edit]
References[edit]
- ↑ 1.0 1.1 J Abraham; P Abreu; M Aglietta; C Aguirre; D Allard; The Pierre Auger Collaboration (November 9, 2007). "Correlation of the highest-energy cosmic rays with nearby extragalactic objects". Science 318 (5852): 938-43. doi:10.1126/science.1151124. Bibcode: 2007Sci...318..938T. http://www.sciencemag.org/content/318/5852/938.full. Retrieved 2013-11-04.
- ↑ 2.0 2.1 Daniele Fargion (April 2010). "UHECR besides CenA: Hints of galactic sources". Progress in Particle and Nuclear Physics 64 (2): 363-5. doi:10.1016/j.ppnp.2009.12.049. Bibcode: 2010PrPNP..64..363F. http://www.sciencedirect.com/science/article/pii/S0146641009001276. Retrieved 2014-01-09.
- ↑ 3.0 3.1 3.2 Bill Keel. Composite emission-line spectrum of NGC 4151. Tucson, Arizona USA: University of Arizona. October 2003 [2014-03-19].
- ↑ 4.0 4.1 4.2 4.3 4.4 4.5 Darach Watson. AGNs as a new standard candle?. Physics Organization. September 27, 2011 [2014-03-27].
- ↑ 5.0 5.1 Tammy Plotner. AGNs as a new standard candle?. Physics Organization. September 27, 2011 [2014-03-27].
- ↑ 6.00 6.01 6.02 6.03 6.04 6.05 6.06 6.07 6.08 6.09 John Biretta. Hubble Detects Faster-Than-Light Motion in Galaxy M87. Baltimore. Maryland USA: Space Telecsope Science Institute. January 6, 1999 [2013-04-28].
- ↑ Duccio Macchetto. Hubble Detects Faster-Than-Light Motion in Galaxy M87. Baltimore. Maryland USA: Space Telecsope Science Institute. January 6, 1999 [2013-04-28].
- ↑ Ann E. Wehrle; Norbert Zacharias; Kenneth Johnston; David Boboltz; Alan L. Fey; Ralph Gaume; Roopesh Ojha; David L. Meier; David W. Murphy; Dayton L. Jones; Stephen C. Unwin; B. Glenn Piner. What is the structure of Relativistic Jets in AGN on Scales of Light Days? In: Galaxies Across Cosmic Time. February 11, 2009 [2013-04-28].
- ↑ Yale University - Office of Public Affairs (2006, June 20). Evidence for Ultra-Energetic Particles in Jet from Black Hole (http://web.archive.org/web/20080513034113/http://www.yale.edu/opa/newsr/06-06-20-01.all.html)
- ↑ Meier, L. M. (2003). The Theory and Simulation of Relativistic Jet Formation: Towards a Unified Model For Micro- and Macroquasars, 2003, New Astron. Rev. , 47, 667. (http://arxiv.org/abs/astro-ph/0312048)
- ↑ Semenov, V.S., Dyadechkin, S.A. and Punsly (2004, August 13). Simulations of Jets Driven by Black Hole Rotation. Science, 305, 978-980. (http://www.sciencemag.org/cgi/content/abstract/sci;305/5686/978?maxtoshow=&HITS=10&hits=10&RESULTFORMAT=&fulltext=relativistic+jet&searchid=1&FIRSTINDEX=10&resourcetype=HWCIT)
- ↑ Georganopoulos, M.; Kazanas, D.; Perlman, E.; Stecker, F. (2005) Bulk Comptonization of the Cosmic Microwave Background by Extragalactic Jets as a Probe of their Matter Content, The Astrophysical Journal , 625, 656. (http://arxiv.org/abs/astro-ph/0502201)
- ↑ BD2412. active galactic nucleus. San Francisco, California: Wikimedia Foundation, Inc. 27 September 2011 [24 July 2018].
- ↑ Długosz. accretion disk. San Francisco, California: Wikimedia Foundation, Inc. 21 April 2004 [25 July 2018].
- ↑ 15.0 15.1 S. Swordy (2001). "The energy spectra and anisotropies of cosmic rays". Space Science Reviews 99: 85–94.
- ↑ A.M. Atoyan (April 1992). "Relativistic neutrons in active galactic nuclei. I-Energy transport from the core. II-Gamma-rays of high and very high energies". Astronomy and Astrophysics 257 (2): 465-75. Bibcode: 1992A&A...257..465A. http://adsabs.harvard.edu/full/1992A%26A...257..465A. Retrieved 2013-10-22.
- ↑ NASA. NASA’s Fermi Probes “Dragons” of the Gamma-ray Sky.
- ↑ Cox, A. N. (編). Allen's Astrophysical Quantities. New York: Springer-Verlag. 2000: 124. ISBN 0-387-98746-0.
- ↑ 19.0 19.1 19.2 19.3 19.4 19.5 19.6 Andrzej A. Zdziarski; Gabriele Ghisellini; Ian M. George; R. Svensson; A. C. Fabian; Chris Done (November 1, 1990). "Electron-positron pairs, Compton reflection, and the X-ray spectra of active galactic nuclei". The Astrophysical Journal 363 (11): L1-4. doi:10.1086/185851. Bibcode: 1990ApJ...363L...1Z. http://adsabs.harvard.edu/full/1990ApJ...363L...1Z. Retrieved 2013-08-15.
- ↑ Greiner J (2000). "Catalog of supersoft X-ray sources". New Astron. 5 (3): 137–41. doi:10.1016/S1384-1076(00)00018-X. Bibcode: 2000NewA....5..137G. http://www.mpe.mpg.de/~jcg/sss/ssscat.html.
- ↑ Belinda J. Wilkes; Gary D. Schmidt; Roc M. Cutri; Himel Ghosh; Dean C. Hines; Brant Nelson; Paul S. Smith (January 10, 2002). "The X-Ray Properties of 2MASS Red Active Galactic Nuclei". The Astrophysical Journal 564 (2): L65-8. doi:10.1086/338908. Bibcode: 2002ApJ...564L..65W. http://iopscience.iop.org/1538-4357/564/2/L65/fulltext/. Retrieved 2013-08-02.
- ↑ 22.00 22.01 22.02 22.03 22.04 22.05 22.06 22.07 22.08 22.09 22.10 M. I. Lister; M. F. Aller; H. D. Aller; D. C. Homan; K. I. Kellermann; Y. Y. Kovalev; A. B. Pushkarev; J. L. Richards et al. (2013). "MOJAVE. X. Parsec-Scale Jet Orientation Variations and Superluminal Motion in AGN". The Astronomical Journal. http://arxiv.org/pdf/1308.2713v1.pdf. Retrieved 2014-03-17.
- ↑ 23.0 23.1 M. Kadler; E. Ros; M. Perucho; Y. Y. Kovalev; D. C. Homan; I. Agudo; K. I. Kellermann; M. F. Aller; H. D. Aller; M. L. Lister; J. A. Zensus. Superluminal Motions in the Jet of 3C 111. West Virginia USA: National Radio Astronomy Observatory. September 23, 2005 [2014-03-17].
- ↑ 24.0 24.1 Bykov, A. M.; Paerels, F. B. S.; Petrosian, V. (February 2008). "Equilibration Processes in the Warm-Hot Intergalactic Medium". Space Science Reviews 134 (1–4): 141–53. doi:10.1007/s11214-008-9309-4. Bibcode: 2008SSRv..134..141B.
- ↑ Reimers, D. (2002). "Baryons in the diffuse intergalactic medium". Space Science Reviews 100 (1/4): 89. doi:10.1023/A:1015861926654. Bibcode: 2002SSRv..100...89R.
- ↑ Anjali Gupta; M. Galeazzi; E. Ursino (May 2010). "Detection and Characterization of the Warm-Hot Intergalactic Medium". Bulletin of the American Astronomical Society 41: 908. Bibcode: 2010AAS...21631808G.
- ↑ http://www.space.com/scienceastronomy/chandra-missing-matter-100511.html
- ↑ https://archive.is/20130202120147/www.skyandtelescope.com/news/93797364.html
- ↑ T. Brown; J. Tumlinson. Dark matter simulation. Washington, DC USA: NASA. June 5, 2012 [2014-02-08].
- ↑ Rojas, Sebastián García. Galaxy NGC 507 · Deep Sky Objects Browser. DSO.
- ↑ Frommert, Hartmut. NGC 507.
- ↑ New General Catalog Objects: NGC 500 - 549.
- ↑ ESO05. The Centre of the Active Galaxy NGC 1097. Paranal: European Southern Observatory. October 17, 2005 [2013-03-15].
- ↑ http://cseligman.com/text/atlas/ngc17.htm#1700
- ↑ 35.0 35.1 35.2 35.3 35.4 35.5 Judy Schmidt. File:Active Nucleus of NGC 3393.png. San Francisco, California: Wikimedia Foundation, Inc. 10 March 2018 [23 July 2018].
- ↑ 36.0 36.1 36.2 36.3 36.4 36.5 ESA/Hubble; NASA. Core of Messier 100 in super high res. ESA/Hubble & NASA. January 16, 2012 [2013-03-14].
- ↑ Panuzzo, P.; Vega, O.; Bressan, A.; Buson, L. et al. (2007). "The Star Formation History of the Virgo Early-Type Galaxy NGC 4435: The Spitzer Mid-Infrared View". The Astrophysical Journal 656 (1): 206–216. doi:10.1086/510147. Bibcode: 2007ApJ...656..206P.
- ↑ The Tail of NGC 4435
- ↑ Cortese, L.; Bendo, G. J.; Isaak, K. G.; Davies, J. I. et al. (2010). "Diffuse far-infrared and ultraviolet emission in the NGC 4435/4438 system: tidal stream or Galactic cirrus?". Monthly Notices of the Royal Astronomical Society: Letters 403 (1): L26–L30. doi:10.1111/j.1745-3933.2009.00808.x. Bibcode: 2010MNRAS.403L..26C.
- ↑ Crook, Aidan C. et al. (February 2007). "Groups of Galaxies in the Two Micron All Sky Redshift Survey". The Astrophysical Journal 655: 790–813. doi:10.1086/510201. Bibcode: 2007ApJ...655..790C.
- ↑ Masahiro Suganuma; Yuzuru Yoshii; Yukiyasu Kobayashi; Takeo Minezaki; Keigo Enya; Hiroyuki Tomita; Tsutomu Aoki; Shintaro Koshida et al. (March 1, 2006). "Reverberation Measurements of the Inner Radius of the Dust Torus in Nearby Seyfert 1 Galaxies". The Astrophysical Journal 639 (1): 46-63. doi:10.1086/499326. Bibcode: 2006ApJ...639...46S. http://iopscience.iop.org/0004-637X/639/1/46/fulltext. Retrieved 2012-08-16.
- ↑ Slavcheva-Mihova, L.; Mihov, B. (February 2011). "Optical multiband surface photometry of a sample of Seyfert galaxies. I. Large-scale morphology and local environment analysis of matched Seyfert and inactive galaxy samples". Astronomy and Astrophysics 526: A43. doi:10.1051/0004-6361/200913243. Bibcode: 2011A&A...526A..43S. See Table 1.
- ↑ Tovmassian, H. M. (August 1966). "On the radio emission from some peculiar galaxies". Australian Journal of Physics 19: 565. doi:10.1071/ph660565. Bibcode: 1966AuJPh..19..565T.
- ↑ Dibai, É. A.; Esipov, V. F.; Pronik, V. I. (February 1968). "The Nucleus of the Seyfert Galaxy NGC 5548". Soviet Astronomy 11: 553. Bibcode: 1968SvA....11..553D.
- ↑ 45.0 45.1 45.2 45.3 45.4 45.5 45.6 45.7 Davide de Martin. Swiftly moving gas streamer eclipses supermassive black hole. Baltimore, Maryland USA: Space Telescope. 19 June 2014 [25 July 2018].
- ↑ 46.0 46.1 46.2 Jelle Kaastra. Swiftly moving gas streamer eclipses supermassive black hole. Baltimore, Maryland USA: Space Telescope. 19 June 2014 [25 July 2018].
- ↑ Gerard Kriss. Swiftly moving gas streamer eclipses supermassive black hole. Baltimore, Maryland USA: Space Telescope. 19 June 2014 [25 July 2018].
- ↑ Massimo Cappi. Swiftly moving gas streamer eclipses supermassive black hole. Baltimore, Maryland USA: Space Telescope. 19 June 2014 [25 July 2018].
- ↑ Pierre-Olivier Petrucci. Swiftly moving gas streamer eclipses supermassive black hole. Baltimore, Maryland USA: Space Telescope. 19 June 2014 [25 July 2018].
- ↑ 50.0 50.1 50.2 50.3 Potw1801a. Lasers and supermassive black holes. 1 January 2018 [1 January 2018].
- ↑ 51.0 51.1 51.2 51.3 M. Machacek. Galaxy Collision Switches on Black Hole. Washington: NASA. 9 October 2012 [21 July 2018].
- ↑ Resonant absorption troughs in the gamma-ray spectra of QSO See section 4.2
- ↑ 53.0 53.1 J. R. Webb; M. T. Carini; S. Clements; S. Fajardo; P. P. Gombola; R. J. Leacock et al. (1990). "The 1987-1990 optical outburst of the OVV quasar 3C 279". Astronomical Journal 100: 1452–1456. doi:10.1086/115609. Bibcode: 1990AJ....100.1452W.
- ↑ APOD: December 26, 1998 - Gamma Ray Quasar
- ↑ Apparent superluminal motion
- ↑ 56.0 56.1 56.2 56.3 56.4 56.5 56.6 Vincent L. Fish; Kazunori Akiyama; Katherine L. Bouman; Andrew A. Chael; Michael D. Johnson; Sheperd S. Doeleman; Lindy Blackburn; John F. C. Wardle et al. (27 October 2016). "Observing—and Imaging—Active Galactic Nuclei with the Event Horizon Telescope". Galaxies 4 (4): 54. doi:10.3390/galaxies4040054. http://www.mdpi.com/2075-4434/4/4/54/pdf. Retrieved 20 July 2018.
- ↑ 57.0 57.1 57.2 57.3 Hubble. From microwaves to megamasers. Washington, DC USA: NASA. September 11, 2017 [23 July 2018].
- ↑ 58.0 58.1 58.2 58.3 58.4 58.5 58.6 Andrew S. Wilson. Circinus Galaxy Spews Gas Into Space. Baltimore, Maryland USA: Hubble Site. 10 April 1999 [23 July 2018].
- ↑ 59.0 59.1 potw1004a. A clump of galaxy misfits. Baltimore, Maryland USA: Space Telescope. 17 May 2010 [22 July 2018].
- ↑ 60.0 60.1 60.2 M. Cisternas. Selected galaxies from the COSMOS survey. Baltimore, Maryland USA: Space Telescope. 5 January 2011 [22 July 2018].
- ↑ Scoville, Nick; Aussel, H.; Brusa, M.; Capak, P.; Carollo, C. Marcella; Elvis, M.; Giavalisco, M.; Guzzo, L. et al. (2007). "The Cosmic Evolution Survey (COSMOS): Overview". The Astrophysical Journal Supplement Series 172: 1. doi:10.1086/516585. Bibcode: 2007ApJS..172....1S.
- ↑ COSMOS. [19 October 2015].
- ↑ 63.0 63.1 63.2 Judy Schmidt. Monster in the deep. Baltimore, Maryland USA: Space Telescope. 30 April 2018 [23 July 2018].
- ↑ 64.0 64.1 64.2 Potw1829a. Major mergers. Baltimore, Maryland USA: Space Telescope. 16 July 2018 [23 July 2018].
- ↑ 65.0 65.1 65.2 J. Comerford. Hubble views a supermassive black hole burping — twice. Baltimore, Maryland USA: Space Telescope. 15 January 2018 [23 July 2018].
- ↑ 66.0 66.1 66.2 66.3 66.4 66.5 66.6 66.7 Rogier Windhorst. GOODS/ERS2 Field. Baltimore, Maryland USA: Hubble Site. January 5, 2010 [23 July 2018].
- ↑ Sam L. VanLandingham (May 2010). "Use of diatoms in determining age and paleoenvironment of the Valsequillo (Hueyatiaco) early man site, Puebla, Mexsico, with corroboration by Chrysophyta cysts for a maximum Yarmouthian (430,000-500,00yr BP) age of the artifacts". Nova Hedwigia 136: 127-38. http://www.pleistocenecoalition.com/vanlandingham/VanLandingham_2010b.pdf. Retrieved 2017-06-16.
- ↑ 68.0 68.1 Paul A. Laviolette (March 1987). "Cosmic-ray volleys from the Galactic Center and their recent impact on the Earth environment". Earth, Moon, and Planets 37 (03): 241-86. doi:10.1007/BF00116639. Bibcode: 1987EM%26P...37..241L. http://adsabs.harvard.edu/full/1987EM%26P...37..241L. Retrieved 2014-09-29.
- ↑ 69.0 69.1 69.2 69.3 D. Alexander. Stacks of Light. Palo Alto, California USA: Caltech. 25 October 2007 [23 July 2018].
- ↑ HA Hoff (August 1983). "EXOSAT - The new extrasolar X-ray observatory". Journal of the British Interplanetary Society (Space Chronicle) 36 (8): 363–7. https://web.archive.org/web/20120829162441/http://md1.csa.com/partners/viewrecord.php?requester=gs&collection=TRD&recid=A8339971AH&q=&uid=788028604&setcookie=yes.
- ↑ 71.0 71.1 71.2 71.3 71.4 Brian G. Taylor. Exosat control. European Space Operations Centre, Darmstadt, Germany: European Space Agency (ESA). 26 May 1983 [23 July 2018].
- ↑ 72.0 72.1 72.2 72.3 72.4 72.5 72.6 Vyacheslav Stepanyuchenko. File:Simeiz RT-22.jpg. San Francisco, California: Wikimedia Foundation, Inc. 8 September 2005 [23 July 2018].
External links[edit]
Template:Principles of radiation astronomy{{Radiation astronomy resources}}