Radiation astronomy/Emissions
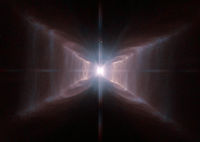
"[T]he extended red emission (ERE) [is] observed in many dusty astronomical environments, in particular, the diffuse interstellar medium of the Galaxy. ... silicon nanoparticles provide the best match to the spectrum and the efficiency requirement of the ERE."[1]
Objects[edit]
"It is possible that the X-ray continuum is primary while the radio and optical emission are secondary for all BL Lac objects when the effect of relativistic beaming is considered. Pair production is a possible mechanism for producing X-ray emissions, while the optical and radio emission would be a consequence of this model (Zdziarski & Lightman 1985; Svensson 1986; Fabian et al. 1986). Barr & Mushotzky (1986) showed a significant correlation between the X-ray luminosity and timescale of X-ray variability for Seyfert galaxies and quasars and interpreted this as evidence that the emitting plasma is near the limit of being dominated by electron-positron pairs."[2]
Continua[edit]
The X-ray continuum can arise from bremsstrahlung, black-body radiation, synchrotron radiation, or what is called inverse Compton scattering of lower-energy photons by relativistic electrons, knock-on collisions of fast protons with atomic electrons, and atomic recombination, with or without additional electron transitions.[3]
"The annihilation of positrons with electrons gives rise to two spectral features, a line emission at 511 keV and a positronium continuum emission (which increases in intensity with energy roughly as a power law up to 511 keV and falls abruptly to zero above 511 keV)[4]."[4]
Absorptions[edit]
"[M]odels in which γ-rays are absorbed in collisions with X-rays producing nonthermal electron-positron pairs, which in turn radiate further X-rays [have been developed]."[5]
"[T]he reprocessing of radiation by e+ e- pairs could be a sufficiently robust mechanism to yield the canonical spectrum, independent of the details of the particle acceleration mechanism and the parameters of the source, such as the X- and γ-ray luminosity, L, and the size, R."[5]
"[T]he hard X-ray spectrum of a growing number of [active galactic nuclei] AGN [in] the 1-30 keV X-ray emission has four distinct components":[5]
- "an incident power law spectrum with a spectral index αix ≃ 0.9,"[5]
- "an emission line at the energy ~6.4 keV (interpreted as a fluorescent iron K-line),"[5]
- "an absorption edge at 7-8 keV (interpreted as an iron K-edge), and"[5]
- "a broad excess of emission with respect to the underlying power law at energies ≳ 10 keV (interpreted as Compton reflection from cold [T < 106 K, optically thick] material)." [5]
Radioactivity emissions[edit]
In Template:SubatomicParticle decay, or "positron emission", the weak interaction converts a nucleus into its next-lower neighbor on the periodic table while emitting an positron (Template:SubatomicParticle) and an electron neutrino (Template:SubatomicParticle):
Template:SubatomicParticle decay cannot occur in an isolated proton because it requires energy due to the mass of the neutron being greater than the mass of the proton. Template:SubatomicParticle decay can only happen inside nuclei when the value of the binding energy of the mother nucleus is less than that of the daughter nucleus. The difference between these energies goes into the reaction of converting a proton into a neutron, a positron and a neutrino and into the kinetic energy of these particles.
Positron emission' or beta plus decay (β+ decay) is a type of beta decay in which a proton is converted, via the weak force, to a neutron, releasing a positron and a neutrino.
Isotopes which undergo this decay and thereby emit positrons include carbon-11, potassium-40, nitrogen-13, oxygen-15, fluorine-18, and iodine-121. As an example, the following equation describes the beta plus decay of carbon-11 to boron-11, emitting a positron and a neutrino:
Gamma rays[edit]
Notation: let the symbol LAT represent Large Area Telescope.
Notation: let the symbol GBM represent Gamma-ray Burst Monitor.
"The observed correlated variability of the GBM and LAT emissions indicates that photons formed co-spatially, with the lower-energy (GBM) photons providing target photons that can interact with higher energy γ rays to produce electron-positron pairs."[6]
X-rays[edit]
X-ray observations of galaxy clusters and groups have discovered a large amount of hot, metal-rich gas.[7]
Clusters of galaxies are the largest bound systems known, with their baryonic mass dominated by X-ray emitting plasma (coronal clouds), which is ten times the mass of the sum of the constituent galaxies.[8]
The "puddles of X-ray emission seen around the centres of galaxies were not the only diffuse X-ray sources. Around the galaxy clusters in Virgo and Coma, puddles measuring nearly 3° across could be seen, and other, more distant, galaxy clusters were accompanied by similar, smaller halos. The familiar optical images of these clusters revealed that they typically contained hundreds to thousands of galaxies, spread over tens of millions of light years, but the X-ray emission showed that there was also some smooth distribution of material in between the galaxies – an intergalactic medium. The significance of its X-ray emission was that for the first time, the amount of gas lying in the vast spaces between galaxies could be estimated, and surprisingly, it seemed that there was ten times as much invisible gas between galaxies as there was within them. This gas was understood to have reached temperatures of tens of millions of degrees in the process of falling in towards the immense gravitational attraction of the galaxy cluster, and hence to have become a source of X-rays."[9]
Synchrotrons[edit]

Alpha decay is characterized by the emission of an alpha particle, a 4He nucleus. The mode of this decay causes the parent nucleus to decrease by two protons and two neutrons. This type of decay follows the relation:
Neutron activation is the process in which neutron radiation induces radioactivity in materials, and occurs when atomic nuclei capture free neutrons, becoming heavier and entering excited states. The excited nucleus often decays immediately by emitting particles such as neutrons, protons, or alpha particles. The neutron capture, even after any intermediate decay, often results in the formation of an unstable activation product. Such radioactive nuclei can exhibit half-lives ranging from small fractions of a second to many years.
Proton emission (also known as proton radioactivity) is a type of radioactive decay in which a proton is ejected from a nucleus. Proton emission can occur from high-lying excited states in a nucleus following a beta decay, in which case the process is known as beta-delayed proton emission, or can occur from the ground state (or a low-lying [nuclear isomer] isomer) of very proton-rich nuclei, in which case the process is very similar to alpha decay.
Beta decay is characterized by the emission of a neutrino and a negatron which is equivalent to an electron. This process occurs when a nucleus has an excess of neutrons with respect to protons, as compared to the stable isobar. This type of transition converts a neutron into a proton; similarly, a positron is released when a proton is converted into a neutron. These decays follows the relation:
Gamma ray emission is follows the previously discussed modes of decay when the decay leaves a daughter nucleus in an excited state. This nucleus is capable of further de-excitation to a lower energy state by the release of a photon. This decay follows the relation:
Generation of electromagnetic radiation can occur whenever charged particles pass within certain distances of each other without being in fixed orbits, the accelerations (or decelerations) may give off the radiation. This is partly illustrated by the diagram at right where an electron has its course altered by near passage by a positive particle. Bremsstrahlung radiation also occurs when two electrons or other similarly charged particles pass close enough to deflect, slow down, or speed up at least one of the particles.
Bremsstrahlung includes synchrotron and cyclotron radiation.
When high-energy radiation bombards materials, the excited atoms within emit characteristic "secondary" (or fluorescent) radiation.
"Direct evidence for in situ particle acceleration mechanisms in the intergalactic medium (IGM) is provided by the diffuse Mpc-scale synchrotron emissions observed from galaxy clusters."[13]
Submillimeters[edit]
The "discovery of the anomalous dust-correlated microwave emission (AME) in the galaxy [was] by Leitch et al (1997) [18] [Characteristics include]
- the AME constitutes a foreground emission to cosmic microwave background (CMB) radiation. [...]
- it provides a window into the properties of small grains, which play crucial roles for the physics and chemistry of the ISM.
- [It is a] diffuse and localized AME"[14]
"In the case of electric dipole radiation, the associated fluctuation in angular momentum is due to absorption of and decays stimulated by microwave photons (dominated by Cosmic Microwave Background (CMB) photons in the diffuse ISM)."[14]
"The [warm ionized medium] WIM is characterized by a large gas temperature T ≈ 8000 K, and a fully ionized gas at low density, nH+ ≈ 0.1 cm-3. Collisions with ions provide the dominant excitation mechanism. Grains are mostly negatively charged due to the high rate of sticking collisions with high-velocity electrons. For a coronene molecule, the characteristic time between ion collisions and the characterstic rotational damping time at the peak angular momentum τrot = √ττed turn out to be comparable6, of order a few years."[14]
The "peak emissivity is enhanced by about 23% for the WIM [and only 11 % for the warm neutral medium (WNM)], although the peak frequency remains unchanged."[14]
"A more important effect on the spectrum is that of increasing the characteristic internal temperature Tω, which makes the grains wobble rather than simply spin about their axis of greatest inertia."[14]
For triaxiality there is an "additional enhancement of the peak frequency and total power by up to the same factors (~ 30 % and 2, respectively) for a large internal relaxation temperature and highly elliptical grains."[14]
"The spectrum includes dust continuum, molecular rotation line and atomic fine-structure line emissions."[15]
"Stretching across almost 100 light-years of space, the Snake nebula is located about 11,700 light-years from Earth in the direction of the constellation Ophiuchus."[16]
"In images from NASA's Spitzer Space Telescope, which observes infrared light, it appears as a sinuous, dark tendril against the starry background. It was targeted because it shows the potential to form many massive stars (stars with more than 8 times the mass of our Sun). SMA was used to observe sub-millimetre radiation from the nebula, radiation emitted between the infrared and radio parts of the electromagnetic spectrum."[16]
"The two panels [at right] show the Snake nebula as photographed by the Spitzer and Herschel space telescopes. At mid-infrared wavelengths (the upper panel taken by Spitzer), the thick nebular material blocks light from more distant stars. At far-infrared wavelengths, however (the lower panel taken by Herschel), the nebula glows due to emission from cold dust. The two boxed regions, P1 and P6, were examined in more detail by the Submillimeter Array."[16]
"To learn how stars form, we have to catch them in their earliest phases, while they're still deeply embedded in clouds of gas and dust, and the SMA is an excellent telescope to do so."[17]
"The team studied two specific spots within the Snake nebula, designated P1 and P6. Within those two regions they detected a total of 23 cosmic "seeds" -- faintly glowing spots that will eventually give birth to between one and a few stars. The seeds generally weigh between 5 and 25 times the mass of the Sun, and each spans a few hundred billion kilometres (for comparison the average Earth-Sun distance is 150 million km). The sensitive, high-resolution SMA images not only unveil the small seeds, but also differentiate them in age."[16]
"Previous theories proposed that high-mass stars form within very massive, isolated "cores" weighing at least 100 times the mass of the Sun. These new results show that that is not the case. The data also demonstrate that massive stars aren't born alone but in groups."[16]
Superluminals[edit]
"Seyfert galaxies were originally noted for the strength and broadening of their emission lines, and as a class were later characterized by the high ionization states of many of the atomic and ionized species producing these lines. This composite spectrum of the archetypal Seyfert NGC 4151 shows the wide variety of emission lines present, from the Lyman limit at 912 A to the mid-infrared at about 9 microns. It uses spectra taken with apertures several arcseconds in size, so as to reproduce the usual spectrum mixing broad and narrow-line components. From 912-1800 A, the data come from the Shuttle-borne Hopkins Ultraviolet Telescope; from 1800-3200 A, from the mean of three measurements by the International Ultraviolet Explorer (IUE) taken at similar brightness levels; from 3200-4000A, from an observation at Kitt Peak National Observatory, with the continuum rescaled to match the adjacent spectra; from 4000-8000 A, a CCD observation obtained at the Lick Observatory 3-m Shane telescope by Alexei Filippenko; from 8000 A to 1 microns, an observation using the same telescope by Donald Osterbrock and collaborators, carefully corrected for atmospheric absorption; from 0.9-2.4 microns, measurements by Rodger Thompson at Steward Observatory's 2,3-m Bok telescope, and on into the infrared, from the Infrared Space Observatory provided by Eckhard Sturm. Because NGC 4151 is irregularly variable, some of the spectral components have been scaled to make the various pieces match for this presentation (so the relative strengths of lines in very different spectral regions may not be accurate)."[18]
"Some of the most prominent emission lines are marked for reference. The permitted lines - those that can be produced at high densities by astronomical standards - show both brad and narrow components. The strongest of these are the hydrogen recombination lines, such as Lyman alpha at 1216 A, H-beta at 4861, and H-alpha at 6563, plus the strong ultraviolet lines of C IV at 1549 and Mg II at 2800. Other features produced only by very rarefied gas at densities of 1000 atoms per cubic centimeter or so - the forbidden lines, denoted by brackets - arise in regions with less velocity structure and are narrower. Some strong examples are [O III] at 4959 and 5007 A, [O II] at 3727, [Ne V] at 3426, and [S III] at 9060 and 9532."[18]
"The spectra of active galactic nuclei are noteworthy in showing species with a large range in ionization at once, from neutral ions such as [O I] and [N I] to highly ionized cases such as [Ne V] and [O VI]. Even hot stars such as light up gaseous nebulae in our galaxy cannot ionize gas as highly as these ions require, so that both a strong source of hard radiation and a wide range in gas density must be present to see such spectra."[18]
Hydrogens[edit]

{{free media}}
The Balmer series of emission lines from hydrogen occur in the visible spectrum of the Sun at: 397, 410, 434, 486, and 656 nm.
Hydrogen has two emission lines that occur in an electron cyclotron resonance (ECR) heated plasmas at 397.007 nm of the Balmer series (Hε) and 434.05 nm Hγ.[19]
Heliums[edit]

As shown in the above spectrum, helium has at least one emission line in the violet.
The He I emission lines are at 414.3 nm and 447.1 nm.[20]
Lithiums[edit]

"Violet satellite bands are caused by those lithium atoms which undergo an optical transition while a helium atom is nearby."[21]
Berylliums[edit]

Above is a light spectrum of the emission and absorption lines of neutral, atomic beryllium. Important for violet astronomy is the apparent absence of strong lines well within the violet range and one strong line on the fringe of the violet and blue portions of the visual spectrum.
Borons[edit]

Above is a light spectrum of the emission and absorption lines of neutral, atomic boron. Important for violet astronomy is the two strong lines well within the violet range and one weaker line on the fringe of the violet and blue portions of the visual spectrum.
The emission and absorption spectra for boron contain lines on the border between violet and blue.
Carbons[edit]

Carbon has an emission line that occurs in plasmas at 449.881 nm from C VI.[19]
From the spectrum above, carbon has at least two lines in the violet.
Nitrogens[edit]

Nitrogen has an emission line that occurs in plasmas at 388.678 nm from N VII.[19]
As seen in its spectrum above, nitrogen has many emission lines in the violet.
Oxygens[edit]

{{free media}}
Oxygen has several emission lines that occur in an electron cyclotron resonance (ECR) heated plasmas: 406.963, 406.99, 407.22, 407.59, 407.89, 408.51, 435.12, 441.489, and 441.697 nm from O II, and 434.045 nm from O VIII.[19]
"Electron temperatures are generally derived from the ratio of auroral to nebular lines in [O III] or [N II]."[22] "[B]ecause of the proximity of strong night-sky lines at λ4358 and λλ5770, 5791, the auroral lines of [O III] λ4363 and [N II] λ5755 are often contaminated."[22]
Fluorines[edit]
{{fairuse}}
Fluorine has an emission line that occurs in plasmas at 429.92 nm from F II.[19]
The emission and absorption spectra of fluorine contains at least eight lines or bands from the cyan to the ultraviolet.[23]
Neons[edit]

{{free media}}
Like fluorine, neon has at least fourteen emission and absorption lines or bands from the cyan to the violet.[24]
Magnesiums[edit]
Magnesium (Mg I) has an absorption band at 416.727±2.9 nm with an excitation potential of 4.33 eV.[25]
Magnesium (Mg II) has an absorption band at 439.059±6.6 nm with an excitation potential of 9.96 eV.[25]
Aluminums[edit]
"The aluminium abundance was derived from the resonance line at 394.4nm, and Al is underabundant by ∼ −0.7 dex with respect to iron."[26] "These abundances are the LTE values; no NLTE corrections, as prescribed by Baumüller and Gehren (1997) and Baumüller et al. (1998), have been applied. The prescribed NLTE corrections for Teff = 6500K, log g = 4.0, [Fe/H] = –3.0 are –0.11 ... for ... Al .... If we assume these values to apply for our lower-gravity star [CS 29497-030], then Al follows iron"[26]. The elemental abundance ratios for CS 29497-030 of aluminum are [Al/H] = -3.37, [Al/Fe] = -0.67.[26]
Both Al I absorption lines at 394.401±8.5 and 396.152±6.5 have been measured for Sirius.[25]
Silicons[edit]
Silicon (Si II) has two absorption bands at 412.805±10.8 nm and 413.088±13.0 nm with excitation potentials of 9.79 eV and 9.80 eV, respectively.[25]
Silicon has an absorption line (Si IV) at 408.9 nm.[20]
Argons[edit]
Argon has several emission lines that occur in an electron cyclotron resonance (ECR) heated plasmas: 426.653, 428.29, 433.12, 434.8064, 437.075, 437.967, 442.60, and 443.019 nm from Ar II.[19]
Calciums[edit]
As of 1977, "model calculations cannot reproduce the observed breadth of the Ca II λ3933 line in Da,F stars like Ross 627 without appealing to an unknown line-broadening mechanism".[27]
Calcium has a line occurring in the solar corona at 408.63 nm of Ca XIII.[28]
Calcium (Ca I) has two absorption bands, 422.673±4.5 nm and 430.253±0.6.[25] The second has an excitation potential of 1.89 eV.[25]
Calcium (Ca II) has an absorption band, 393.366±55.0.[25]
Scandiums[edit]
Scandium (Sc II) has an absorption band, 424.683±1.0 nm, with an excitation potential of 0.31 eV.[25]
Titaniums[edit]
Titanium (Ti II) has an absorption band, 391.346-441.108 nm, with an excitation potential range of 0.60-3.08 eV.[25]
Vanadiums[edit]

The chemistry of vanadium is noteworthy for the accessibility of the four adjacent oxidation states 2-5. In aqueous solution the colours are lilac V2+(aq), green V3+(aq), blue VO2+(aq) and, at high pH, yellow VO42-.
Vanadium (V II) has an absorption band, 392.973-403.678 nm, with an excitation potential range of 1.07-1.81 eV.[25]
Chromiums[edit]
Chromium (Cr) has emission lines that occur in plasmas at 425.435, 427.48, and 428.972 nm from Cr I.[19]
Chromium has absorption lines that occur at 425.435-428.972 nm from Cr I and 400.333-428.421 nm, 3.09-6.46 eV from Cr II near Sirius.[25]
Manganeses[edit]
Manganese (Mn I) has two absorption bands at 403.449±1.4 nm and 405.554±0.8 nm, where the second has an excitation potential of 2.13 eV.[25]
Manganese (Mn II) has an absorption band at 420.638±0.8 nm with an excitation potential of 5.37 eV.[25]
Irons[edit]

{{free media}}
Iron has a line occurring in the solar corona in the violet at 398.69 nm of Fe XI.[28]
Iron (Fe I) has an absorption band at 392.291-440.475 nm with an excitation potential range of 0.05-2.46 eV.[25]
Iron (Fe II) has an absorption band at 393.829-438.538 nm with an excitation potential range of 1.66-2.77 eV.[25]
Iron (Fe II) has an emission line at 489.1 nm.[29]
The Fraunhofer G line at 430.790 nm is from iron.
Nickels[edit]
Nickel has three emission lines occurring in the solar corona at 380.08 nm of Ni XIII and 423.14 nm and 431.1 of Ni XII.[28]
Nickel has an absorption band at 401.550-436.210 nm with an excitation potential of 4.01 eV.[25]
Strontiums[edit]
Strontium (Sr II) has two absorption bands: 407.771±11.3 nm and 421.552±10.4 nm.[25]
Yttriums[edit]
Yttrium (Y II) has an absorption band from 395.035 to 439.802 nm, with an excitation potential range of 0.10-0.13 eV.[25]
Zirconiums[edit]
Zirconium (Zr II) has an absorption band, 395.824-415.624 nm, with an excitation potential of 0.52-0.75 eV.[25]
Mercuries[edit]

{{free media}}
As the spectrum of mercury (Hg) indicates, it has lines in the violet.
Cyanides[edit]
"The CN radical through emission in its Violet system bands has a long-established presence in comets."[30]
Hydrogen sulfide emissions[edit]
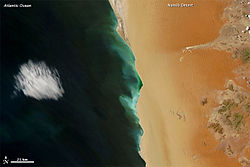
In the image at right, "[p]ale green patterns tinted the water along the Namibian coast in late February 2012. But unlike other bright hues that occasionally show up in the ocean, these colors didn’t result from a phytoplankton bloom.[31]
"[H]ydrogen sulfide gas is emitted periodically along the Namibian coast. Ocean currents carry oxygen-poor water to the region, and chemical and biological processes can deplete what little oxygen is available. The sediments in the local seafloor are also rich with organic matter. When organic matter decays in an oxygen-poor environment, hydrogen sulfide emissions can result."[31]
"The Moderate Resolution Imaging Spectroradiometer (MODIS) on NASA’s Terra satellite captured this natural-color image on February 29, 2012. The pale-hued surface waters snake along the shore of the Namib Desert, stretching roughly 150 kilometers (90 miles)."[31]
"The milky-green colors along Namibia’s coast indicate high concentrations of sulfur and low concentrations of oxygen. Episodes like this aren’t just colorful, they are actually toxic to local marine organisms. Fish die in the low-oxygen water; however, what is deadly for the fish can be good for birds that feed on their carcasses. Likewise, lobsters crawling onto shore to escape the toxic seawater can make meals for locals. And some species of foraminifera—tiny shelled marine organisms—actually thrive in the oxygen-poor sea floor sediments off the Namibian coast."[31]
Tephras[edit]
Def. the solid material thrown into the air by a volcanic eruption that settles on the surrounding areas is called tephra.
"[T]ephra, is a general term for fragments of volcanic rock and lava that are blasted into the air by volcanic explosions or carried upward in the volcanic plume by hot, hazardous gases. The larger fragments usually fall close to the volcano, but the finer particles can be advected quite some distance. ... [Fine ash] can contain rock, minerals, and volcanic glass fragments smaller than .1 inch in diameter, or slightly larger than the size of a pinhead."[32]
Coronal clouds[edit]

The solar flare at Active Region 10039 on July 23, 2002, exhibits many exceptional high-energy phenomena including the 2.223 MeV neutron capture line and the 511 keV electron-positron (antimatter) annihilation line. In the image at right, the RHESSI low-energy channels (12-25 keV) are represented in red and appear predominantly in coronal loops. The high-energy flux appears as blue at the footpoints of the coronal loops. Violet is used to indicate the location and relative intensity of the 2.2 MeV emission.
During solar flares “[s]everal radioactive nuclei that emit positrons are also produced; [which] slow down and annihilate in flight with the emission of two 511 keV photons or form positronium with the emission of either a three gamma continuum (each photon < 511 keV) or two 511 keV photons."[33] The Reuven Ramaty High Energy Solar Spectroscopic Imager (RHESSI) made the first high-resolution observation of the solar positron-electron annihilation line during the July 23, 2003 solar flare.[33] The observations are somewhat consistent with electron-positron annihilation in a quiet solar atmosphere via positronium as well as during flares.[33] Line-broadening is due to "the velocity of the positronium."[33] "The width of the annihilation line is also consistent ... with thermal broadening (Gaussian width of 8.1 ± 1.1 keV) in a plasma at 4-7 x 105 K. ... The RHESSI and all but two of the SMM measurements are consistent with densities ≤ 1012 H cm-3 [but] <10% of the p and α interactions producing positrons occur at these low densities. ... positrons produced by 3He interactions form higher in the solar atmosphere ... all observations are consistent with densities > 1012 H cm-3. But such densities require formation of a substantial mass of atmosphere at transition region temperatures."[33]
Io[edit]

"The camera on Cassini captured images of eclipsed Io in several colors ranging from the near-ultraviolet to the near-infrared. A black-and-white movie clip of 48 clear-filter frames spanning two hours during the eclipse was released on February 5 (PIA02882). Here, two colors have been added to show the type of evidence used by imaging scientists in determining the source of Io's auroral glows. The color pictures were taken at lower resolution -- 120 kilometers (75 miles) per pixel rather than 60 kilometers(37 miles) per pixel -- and less frequently than the clear-filter images. White dots near the equator are volcanoes, some of which are much brighter than the faint atmospheric glows. The brightest of them is the volcano Pele."[34]
"Emissions of light (at wavelengths of 595 to 645 nanometers) likely arise from a tenuous atmosphere of oxygen. These glows would appear red to the eye and are consequently colored red in the movie. Emissions in near-ultraviolet wavelengths (between 300 and 380 nanometers), corresponding wavelength to the bright blue visible glows one would expect from sulfur dioxide. They have been colored blue in the movie. The blue glows are restricted to areas deep down in the atmosphere near the surface of Io, while the red glows are much more extensive, reaching heights of up to 900 kilometers (560 miles). This would be expected if the blue glows are indeed produced by sulfur dioxide, since sulfur dioxide molecules are heavier than oxygen atoms, so are more closely bound to the surface by gravity. The prominent blue and red regions near the equator of Io dance across the moon with the changing orientation of Jupiter's magnetic field, illustrating the relationship between Io's auroras and the electric currents that excite them."[34]
Gases on Io[edit]

{{free media}}
At right is an "eerie view of Jupiter's moon Io in eclipse ... acquired by NASA's Galileo spacecraft while the moon was in Jupiter's shadow. Gases above the satellite's surface produced a ghostly glow that could be seen at visible wavelengths (red, green, and violet). The vivid colors, caused by collisions between Io's atmospheric gases and energetic charged particles trapped in Jupiter's magnetic field, had not previously been observed. The green and red emissions are probably produced by mechanisms similar to those in Earth's polar regions that produce the aurora, or northern and southern lights. Bright blue glows mark the sites of dense plumes of volcanic vapor, and may be places where Io is electrically connected to Jupiter."[35]
"North is to the top of the picture, and Jupiter is towards the right. The resolution is 13.5 kilometers (8 miles) per picture element. The images were taken on May 31, 1998 at a range of 1.3 million kilometers (800,000 miles) by Galileo's onboard solid state imaging camera system during the spacecraft's 15th orbit of Jupiter."[35]
Volcanic gases[edit]

{{free media}}
Water vapour is consistently the most common volcanic gas, normally comprising more than 60% of total emissions. Carbon dioxide typically accounts for 10 to 40% of emissions.[36]
Volcanoes located at convergent plate boundaries emit more water vapor and chlorine, higher H2O/H2, H2O/CO2, CO2/He and N2/He ratios than volcanoes at geologic hot spots or divergent plate boundaries per the addition of seawater into magmas formed at subduction zones.[36]
In volcanoes with an open path to the surface, e.g. Stromboli in Italy, bubbles may reach the surface and as they pop small explosions occur, where the gas can flow rapidly through the continuous permeable network towards the surface, which explains activity at Santiaguito, Santa Maria volcano, Guatemala[37] and Soufrière Hills Volcano, Montserrat.[38]
Volcanic gases were directly responsible for approximately 3% of all volcano-related deaths of humans between 1900 and 1986.[36]
The greenhouse gas, carbon dioxide, is emitted from volcanoes, accounting for nearly 1% of the annual global total.[39]
Some volcanic gases including sulfur dioxide, hydrogen chloride, hydrogen sulfide and hydrogen fluoride react with other atmospheric particles to form aerosols.[36]
Nebulas[edit]

{{free media}}

{{free media}}
{{fairuse}}
{{fairuse}}
Def. a "nebula composed of ionized gases which produce visible light"[40] is called an emission nebula.
"A wide-field image in the region of NGC 3603 [is] taken on the ground by the Digitized Sky Survey 2. The glowing clouds of hydrogen gas (seen here in orange) compose a vast emission nebula. The field of view is approximately 2.6 x 2.8 degrees."[41]
"The star cluster Pismis 24 lies in the core of the large emission nebula NGC 6357 [in the image on the right] that extends one degree on the sky in the direction of the Scorpius constellation. Part of the nebula is ionised by the youngest (bluest) heavy stars in Pismis 24. The intense ultraviolet radiation from the blazing stars heats the gas surrounding the cluster and creates a bubble in NGC 6357. The presence of these surrounding gas clouds makes probing into the region even harder."[42]
"Stretching across almost 100 light-years of space, the Snake nebula is located about 11,700 light-years from Earth in the direction of the constellation Ophiuchus."[16]
"In images from NASA's Spitzer Space Telescope, which observes infrared light, it appears as a sinuous, dark tendril against the starry background. It was targeted because it shows the potential to form many massive stars (stars with more than 8 times the mass of our Sun). SMA was used to observe sub-millimetre radiation from the nebula, radiation emitted between the infrared and radio parts of the electromagnetic spectrum."[16]
"The two panels [at right] show the Snake nebula as photographed by the Spitzer and Herschel space telescopes. At mid-infrared wavelengths (the upper panel taken by Spitzer), the thick nebular material blocks light from more distant stars. At far-infrared wavelengths, however (the lower panel taken by Herschel), the nebula glows due to emission from cold dust. The two boxed regions, P1 and P6, were examined in more detail by the Submillimeter Array."[16]
"To learn how stars form, we have to catch them in their earliest phases, while they're still deeply embedded in clouds of gas and dust, and the SMA is an excellent telescope to do so."[43]
"The team studied two specific spots within the Snake nebula, designated P1 and P6. Within those two regions they detected a total of 23 cosmic "seeds" -- faintly glowing spots that will eventually give birth to between one and a few stars. The seeds generally weigh between 5 and 25 times the mass of the Sun, and each spans a few hundred billion kilometres (for comparison the average Earth-Sun distance is 150 million km). The sensitive, high-resolution SMA images not only unveil the small seeds, but also differentiate them in age."[16]
"Previous theories proposed that high-mass stars form within very massive, isolated "cores" weighing at least 100 times the mass of the Sun. These new results show that that is not the case. The data also demonstrate that massive stars aren't born alone but in groups."[16]
The image second down on the left is a Hubble Telescope image using an infrared spectrometer. The Pistol "nebula of the insanely bright Pistol star is warped".[44]
"Dust in the Pistol star’s nebula is brilliant, compressed, externally heated and ionized, thanks to its proximity to neighbors in the Quintuplet Cluster."[45]
“The initial attention draw to the Pistol star was its high luminosity, [but] the nebulae around it and its sister star [LBV3] have turned out to be quite interesting. While the Pistol star is a member of the Quintuplet Cluster – although on the outskirts of the cluster – it is about six light-years away from the cluster’s center. That’s about 1.5 times the distance from our solar system to the nearest star.”[46]
“It’s impressive that even at this distance, the rest of the Quintuplet Cluster exerts a large influence on the Pistol nebula.”[46]
References[edit]
- ↑ Adolf N. Witt; Karl D. Gordon; Douglas G. Furton (July 1, 1998). "Silicon Nanoparticles: Source of Extended Red Emission?". The Astrophysical Journal Letters 501 (1): L111-5. doi:10.1086/311453. http://iopscience.iop.org/1538-4357/501/1/L111. Retrieved 2013-07-30.
- ↑ G. Z. Xie; B. F. Liu; J. C. Wang (November 20, 1995). "A Signature of Relativistic Electron-Positron Beams in BL Lacertae Objects". The Astrophysical Journal 454 (11): 50-4. doi:10.1086/176463. Bibcode: 1995ApJ...454...50X. http://adsabs.harvard.edu/full/1995ApJ...454...50X. Retrieved 2013-08-13.
- ↑ P Morrison (1967). "Extrasolar X-ray Sources". Annual Review of Astronomy and Astrophysics 5 (1): 325–50. doi:10.1146/annurev.aa.05.090167.001545. Bibcode: 1967ARA&A...5..325M.
- ↑ P.A. Milne; J.D. Kurfess; R.L. Kinzer; M.D. Leising (July 2002). "Supernovae and Positron Annihilation Radiation". New Astronomy Reviews 46 (8-10): 553-8. http://www.sciencedirect.com/science/article/pii/S1387647302002002. Retrieved 2013-08-13.
- ↑ 5.0 5.1 5.2 5.3 5.4 5.5 5.6 Andrzej A. Zdziarski; Gabriele Ghisellini; Ian M. George; R. Svensson; A. C. Fabian; Chris Done (November 1, 1990). "Electron-positron pairs, Compton reflection, and the X-ray spectra of active galactic nuclei". The Astrophysical Journal 363 (11): L1-4. doi:10.1086/185851. Bibcode: 1990ApJ...363L...1Z. http://adsabs.harvard.edu/full/1990ApJ...363L...1Z. Retrieved 2013-08-15.
- ↑ AA Abdo; M Ackermann; M Arimoto; K Asano; The Fermi LAT; Fermi GBM Collaborations (March 27, 2009). "Fermi observations of high-energy gamma-ray emission from GRB 080916C". Science 323 (5922): 1688-93. doi:10.1126/science.1169101. http://www.sciencemag.org/content/323/5922/1688.short. Retrieved 2013-08-13.
- ↑ Fields BD; Mathews GJ; Scramm DN (July 1997). "Halo white dwarfs and the hot intergalactic medium". The Astrophysical Journal 483 (2): 625-37. doi:10.1086/304291. Bibcode: 1997ApJ...483..625F.
- ↑ White NE; Bookbinder JA; Tananbaum H (2001). "The Constellation X-ray Mission, In: X-ray Astronomy 2000". ASP Conference Proceeding 234: 597-610. Bibcode: 2001ASPC..234..597W.
- ↑ SE London; Roger Pickard; Ron Johnson; Hazel Collett; Nick James (May 28, 2008). Ordinary Meeting, 2008 May 28. http://dcford.org.uk/baapdf/baa20080528.pdf. Retrieved 2014-01-09.
- ↑ http://library.thinkquest.org/27954/dequ.htm
- ↑ http://chemteam.info/Radioactivity/Writing-Alpha-Beta.html
- ↑ Loveland, W., Morrissey, D. J., Seaborg, G. T., Modern Nuclear Chemistry, 2006, John Wiley & Sons, 221.
- ↑ G. Brunetti, A. Lazarian (April 2011). "Particle reacceleration by compressible turbulence in galaxy clusters: effects of a reduced mean free path". Monthly Notices of the Royal Astronomical Society 412 (2): 817-24. doi:10.1111/j.1365-2966.2010.17937.x. Bibcode: 2011MNRAS.412..817B. http://onlinelibrary.wiley.com/doi/10.1111/j.1365-2966.2010.17937.x/full. Retrieved 2014-02-09.
- ↑ 14.0 14.1 14.2 14.3 14.4 14.5 Yacine Ali-Haïmoud (2013). "Spinning dust radiation: a review of the theory". Advances in Astronomy 2013 (462697). doi:10.1155/2013/462697. Bibcode: 2013AdAst2013E...2A. http://arxiv.org/pdf/1211.2748v1.pdf. Retrieved 2014-10-19.
- ↑ T.G. Phillips, J. Keene (November 1992). "Submillimeter astronomy [heterodyne spectroscopy"]. Proceedings of the IEEE 80 (11): 1662-78. doi:10.1109/5.175248. http://ieeexplore.ieee.org/xpls/abs_all.jsp?arnumber=175248. Retrieved 2013-10-21.
- ↑ 16.00 16.01 16.02 16.03 16.04 16.05 16.06 16.07 16.08 16.09 Jean-Charles Cuillandre. Image of the Day: The Snake Nebula --From Cosmic Seeds to Massive Stars. Daily Galaxy. February 26, 2014 [2014-03-13]. Cite error: Invalid
<ref>
tag; name "Cuillandre" defined multiple times with different content - ↑ Ke Wang. Image of the Day: The Snake Nebula --From Cosmic Seeds to Massive Stars. Daily Galaxy. February 26, 2014 [2014-03-13].
- ↑ 18.0 18.1 18.2 Bill Keel. Composite emission-line spectrum of NGC 4151. Tucson, Arizona USA: University of Arizona. October 2003 [2014-03-19].
- ↑ 19.0 19.1 19.2 19.3 19.4 19.5 19.6 K. J. McCarthy; A. Baciero; B. Zurro; TJ-II Team. Impurity Behaviour Studies in the TJ-II Stellarator, In: 27th EPS Conference on Contr. Fusion and Plasma Phys.. Budapest: ECA. June 12, 2000: 1244–7 [2013-01-20].
- ↑ 20.0 20.1 Peter S. Conti; Eva M. Leep (October 1974). "Spectroscopic observations of O-type stars. V. The hydrogen lines and lambda 4686 He II". The Astrophysical Journal 193 (10): 113-24. doi:10.1086/153135. Bibcode: 1974ApJ...193..113C.
- ↑ G. D. Mahan (April 24, 1972). "Violet satellite bands in the spectra of Li perturbed by He". Physics Letters A 39 (2): 145-6. doi:10.1016/0375-9601(72)91056-0. http://www.sciencedirect.com/science/article/pii/0375960172910560. Retrieved 2013-03-23.
- ↑ 22.0 22.1 S. A. Hawley (September 1, 1978). "The chemical composition of galactic and extragalactic H II regions". The Astrophysical Journal 224 (9): 417-36. doi:10.1086/156389. Bibcode: 1978ApJ...224..417H.
- ↑ Alex Petty. Fluorine light signature. alexpetty.com. July 2007 [2013-06-01].
- ↑ Alex Petty. Neon light signature. alexpetty.com. July 2011 [2013-06-01].
- ↑ 25.00 25.01 25.02 25.03 25.04 25.05 25.06 25.07 25.08 25.09 25.10 25.11 25.12 25.13 25.14 25.15 25.16 25.17 25.18 Cite error: Invalid
<ref>
tag; no text was provided for refs namedSadakane
- ↑ 26.0 26.1 26.2 T. Sivarani; P. Bonifacio; P. Molaro; R. Cayrel; M. Spite; F. Spite; B. Plez; J. Andersen et al. (January 2004). "First stars IV. CS 29497-030: Evidence for operation of the s-process at very low metallicity". Astronomy and Astrophysics 413 (1): 1073-85. doi:10.1051/0004-6361:20031590. Bibcode: 2004A&A...413.1073S. http://arxiv.org/pdf/astro-ph/0310291.pdf. Retrieved 2012-06-02.
- ↑ H. L. Shipman (April 1977). "Masses, radii, and model atmospheres for cool white-dwarf stars". The Astrophysical Journal 213 (4): 138-44. doi:10.1086/155138. Bibcode: 1977ApJ...213..138S.
- ↑ 28.0 28.1 28.2 P. Swings (July 1943). "Edlén's Identification of the Coronal Lines with Forbidden Lines of Fe X, XI, XIII, XIV, XV; Ni XII, XIII, XV, XVI; Ca XII, XIII, XV; a X, XIV". The Astrophysical Journal 98 (07): 116-28. doi:10.1086/144550. Bibcode: 1943ApJ....98..116S.
- ↑ Cite error: Invalid
<ref>
tag; no text was provided for refs namedSmith05
- ↑ S.R. Federman, David L. Lambert (May 2002). "The need for accurate oscillator strengths and cross sections in studies of diffuse interstellar clouds and cometary atmospheres". Journal of Electron Spectroscopy and Related Phenomena 123 (2-3): 161-71. http://www.sciencedirect.com/science/article/pii/S0368204802000178. Retrieved 2013-01-20.
- ↑ 31.0 31.1 31.2 31.3 Michon Scott. Hydrogen Sulfide Emissions off of Africa. NASA Earth Observatory. February 28, 2002 [2012-07-22].
- ↑ Mark R. Mireles; Kirth L. Pederson; Charles H. Elford. Meteorologial Techniques. Offutt Air Force Base, Nebraska, USA: Air Force Weather Agency/DNT. February 21, 2007 [2013-02-17].
- ↑ 34.0 34.1 Sue Lavoie. PIA03450: Io Color Eclipse Movie. Tucson, Arizona USA: NASA/JPL/University of Arizona. May 31, 2001 [2013-05-30].
- ↑ 35.0 35.1 Sue Lavoie. PIA01637: Io's Aurorae. Pasadena, California: NASA and the Jet Propulsion Laboratory, California Institute of Technology. October 13, 1998 [2012-07-22].
- ↑ 36.0 36.1 36.2 36.3 H. Sigurdsson et al. (2000) Encyclopedia of Volcanoes, San Diego, Academic Press
- ↑ Holland et al. (2011), Degassing processes during lava dome growth: Insights from Santiaguito lava dome, Guatemala, Journal of Volcanology and Geothermal Research vol. 202 p153-166
- ↑ Hautmann et al. (2014), Strain field analysis on Montserrat (W.I.) as a tool for assessing permeable flow paths in the magmatic system of Soufrière Hills Volcano, Geochemistry, Geophysics, Geosystems vol. 15 p676-690
- ↑ Royal Society Climate Change Controversies, London, June 2007
- ↑ Silent Sam. emission nebula. San Francisco, California: Wikimedia Foundation, Inc. 7 April 2014 [2015-09-26].
- ↑ Davide De Martin. Extreme star cluster bursts into life (ground-based image). Baltimore, Maryland USA: Space Telescope. October 2, 2007 [2014-03-04].
- ↑ Jesús Maíz Apellániz. Star on a Hubble diet. Baltimore, Maryland USA: Space Telescope. 11 December 2006 [2015-09-26].
- ↑ Ke Wang. Image of the Day: The Snake Nebula --From Cosmic Seeds to Massive Stars. Daily Galaxy. February 26, 2014 [2014-03-13].
- ↑ Ryan M. Lau. Pushy Neighbors Force Stellar Twins to Diverge. Astro Watch. 15 April 2014 [2015-09-29].
- ↑ cornell.edu. Pushy Neighbors Force Stellar Twins to Diverge. Astro Watch. 15 April 2014 [2015-09-29].
- ↑ 46.0 46.1 Terry Herter. Pushy Neighbors Force Stellar Twins to Diverge. Astro Watch. 15 April 2014 [2015-09-29].